Nano-alphabet: quantum dots. Quantum dots - nanoscale sensors for medicine and biology
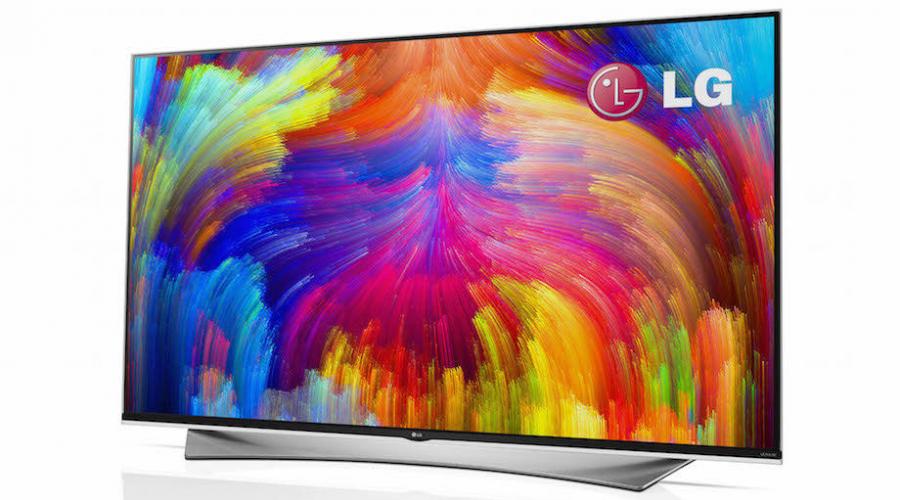
Read also
In order to receive general idea about the properties of material objects and the laws in accordance with which the macroworld familiar to everyone “lives”, it is not at all necessary to complete higher education educational institution, because every day everyone faces their manifestations. Although recently the principle of similarity has been increasingly mentioned, its proponents claim that the micro and macro worlds are very similar, nevertheless, there is still a difference. This is especially noticeable with very small sizes of bodies and objects. Quantum dots, sometimes called nanodots, are one of these cases.
Less less
Let's remember the classical structure of an atom, for example, hydrogen. It includes a nucleus, which, due to the presence of a positively charged proton in it, has a plus, that is, +1 (since hydrogen is the first element in the periodic table). Accordingly, at a certain distance from the nucleus there is an electron (-1), forming an electron shell. Obviously, if you increase the value, this will entail the addition of new electrons (remember: in general, the atom is electrically neutral).
The distance between each electron and the nucleus is determined by the energy levels of the negatively charged particles. Each orbit is constant, the total configuration of the particles determines the material. Electrons can jump from one orbit to another, absorbing or releasing energy through photons of one frequency or another. The most distant orbits contain electrons with the maximum energy level. Interestingly, the photon itself exhibits a dual nature, being defined simultaneously as a massless particle and electromagnetic radiation.
The word “photon” itself is of Greek origin and means “particle of light.” Therefore, it can be argued that when an electron changes its orbit, it absorbs (emits) a quantum of light. IN in this case It is appropriate to explain the meaning of another word - “quantum”. In fact, there is nothing complicated. The word comes from the Latin “quantum”, which literally translates as the smallest value of any physical quantity (here radiation). Let us explain with an example what a quantum is: if, when measuring weight, the smallest indivisible quantity was a milligram, then it could be called that. This is how a seemingly complex term is simply explained.
Quantum Dots Explained
Often in textbooks you can find the following definition for a nanodot - this is an extremely small particle of any material, the dimensions of which are comparable to the emitted wavelength of an electron (the full spectrum covers the limit from 1 to 10 nanometers). Inside it, the value of a single negative charge carrier is less than outside, so the electron is limited in its movements.
However, the term "quantum dots" can be explained differently. An electron that has absorbed a photon “rises” to a higher energy level, and in its place a “shortage” is formed - a so-called hole. Accordingly, if an electron has a -1 charge, then a hole has a +1 charge. Trying to return to its previous stable state, the electron emits a photon. The connection of charge carriers “-” and “+” in this case is called an exciton and in physics is understood as a particle. Its size depends on the level of absorbed energy (higher orbit). Quantum dots are precisely these particles. The frequency of energy emitted by an electron directly depends on the particle size of a given material and exciton. It is worth noting that the color perception of light by the human eye is based on different
LED, LCD, OLED, 4K, UHD... it would seem that the last thing the television industry needs right now is another technical abbreviation. But progress cannot be stopped, meet a couple more letters - QD (or Quantum Dot). Let me immediately note that the term “quantum dots” in physics has a broader meaning than is required for televisions. But in light of the current fashion for everything nanophysical, marketers large corporations happily began to apply this difficult scientific concept. So I decided to figure out what kind of quantum dots these are and why everyone would want to buy a QD TV.
First, some science in a simplified form. A “quantum dot” is a semiconductor whose electrical properties depend on its size and shape (wiki). It must be so small that quantum size effects are pronounced. And these effects are regulated by the size of this very point, i.e. the energy of an emitted, for example, photon - in fact, the color - depends on the “dimensions”, if this word is applicable to such small objects.

In even more consumer language, these are tiny particles that will begin to glow in a certain spectrum if illuminated. If they are applied and “rubbed” on a thin film, then illuminated, the film will begin to luminesce brightly. The essence of the technology is that the size of these dots is easy to control, which means achieving accurate color.

In fact, it is not so important what name large corporations choose, the main thing is what it should give to the consumer. And here the promise is quite simple - improved color rendition. To better understand how “quantum dots” will provide this, you need to remember the design of the LCD display.
Light under the crystal
An LCD TV (LCD) consists of three main parts: a white backlight, color filters (separating the light into red, blue and green colors) and liquid crystal matrix. The latter looks like a grid of tiny windows - pixels, which, in turn, consist of three subpixels (cells). Liquid crystals, like blinds, can block the light flow or, on the contrary, open completely; there are also intermediate states.

When White light, emitted by light-emitting diodes (LED, today it is already difficult to find a TV with fluorescent lamps, as it was just a few years ago), passes, for example, through a pixel whose green and red cells are closed, then we see blue. The degree of “participation” of each RGB pixel changes, and thus a color image is obtained.

As you understand, to ensure the color quality of an image, at least two things are required: accurate filter colors and the correct white backlight, preferably with a wide spectrum. It’s with the latter that LEDs have a problem.
Firstly, they are not actually white, in addition, they have a very narrow color spectrum. That is, the spectrum is wide white is achieved by additional coatings - there are several technologies, most often the so-called phosphor diodes with the addition of yellow are used. But this “quasi-white” color still falls short of the ideal. If you pass it through a prism (like in a physics lesson at school), it will not decompose into all the colors of the rainbow of equal intensity, as happens with sunlight. Red, for example, will appear much dimmer than green and blue.

Engineers, understandably, are trying to correct the situation and come up with workarounds. For example, you can lower the green and blue levels in the TV settings, but this will affect the overall brightness - the picture will become paler. So all manufacturers were looking for a source of white light, the decay of which would produce a uniform spectrum with colors of the same saturation. This is where quantum dots come to the rescue.
Quantum dots
Let me remind you that if we are talking about televisions, then “quantum dots” are microscopic crystals that luminesce when light hits them. They can “burn” in many ways various colors, it all depends on the size of the point. And given that scientists have now learned to control their sizes almost perfectly by changing the number of atoms they consist of, it is possible to obtain a glow of exactly the color you need. Quantum dots are also very stable - they do not change, which means that a dot designed to luminesce at a certain shade of red will remain that shade almost forever.

The engineers came up with the idea of using the technology in the following way: a “quantum dot” coating is applied to a thin film, created to glow with a certain shade of red and green. And the LED is regular blue. And then someone will immediately guess: “everything is clear - there is a source of blue, and the dots will give green and red, which means we will get the same RGB model!” But no, technology works differently.
It must be remembered that “quantum dots” are located on one large sheet and they are not divided into subpixels, but simply mixed together. When a blue diode shines on the film, the dots emit red and green, as mentioned above, and only when all three of these colors are mixed do they create an ideal white light source. And let me remind you that high-quality white light behind the matrix is actually equal to the natural color rendering for the viewer’s eyes on the other side. At a minimum, because you don’t have to make corrections for loss or distortion of the spectrum.
It's still an LCD TV
The wide color gamut will be especially useful for new 4K TVs and 4:4:4 color subsampling, which awaits us in future standards. That's all well and good, but remember that quantum dots don't solve other problems with LCD TVs. For example, it is almost impossible to get perfect black, because liquid crystals (the same “blinds” that I wrote about above) are not able to completely block light. They can only “cover themselves”, but not close completely.
Quantum dots are designed to improve color reproduction, and this will significantly improve the impression of the picture. But this is not OLED technology or plasma, where the pixels are able to completely stop the flow of light. Nevertheless plasma TVs have retired and OLEDs are still too expensive for most consumers, so it's still good to know what manufacturers will be offering us soon the new kind LED TVs, which will show better.
How much does a “quantum TV” cost?
The first QD TVs from Sony, Samsung and LG are promised to be shown at CES 2015 in January. However, China's TLC Multimedia is ahead of the curve, they have already released a 4K QD TV and say it is about to hit stores in China.

On this moment name the exact cost of TVs with new technology impossible, we are waiting for official statements. They wrote that QDs will cost three times less than OLEDs with similar functionality. In addition, the technology, as scientists say, is very inexpensive. Based on this, we can hope that Quantum Dot models will be widely available and simply replace conventional ones. However, I think that prices will still increase at first. As is usually the case with all new technologies.
Good day, Habrazhiteliki! I think many people have noticed that advertisements about displays based on quantum dot technology, the so-called QD – LED (QLED) displays, have begun to appear more and more often, despite the fact that at the moment this is just marketing. Similar to LED TV and Retina, this is a technology for creating LCD displays, using LEDs based on quantum dots as backlight.
Your humble servant decided to figure out what quantum dots are and what they are used with.
Instead of introducing
Quantum dot- a fragment of a conductor or semiconductor, the charge carriers of which (electrons or holes) are limited in space in all three dimensions. The size of the quantum dot must be so small that quantum effects were significant. This is achieved if the kinetic energy of the electron is noticeably greater than all other energy scales: first of all more temperature, expressed in energy units. Quantum dots were first synthesized in the early 1980s by Alexei Ekimov in a glass matrix and by Louis E. Brous in colloidal solutions. The term "quantum dot" was coined by Mark Reed.The energy spectrum of a quantum dot is discrete, and the distance between stationary energy levels of the charge carrier depends on the size of the quantum dot itself as - h/(2md^2), where:
- h - reduced Planck constant;
- d is the characteristic size of the point;
- m is the effective mass of an electron at a point
For example, when an electron moves to a lower energy level, a photon is emitted; Since you can adjust the size of a quantum dot, you can also change the energy of the emitted photon, and therefore change the color of the light emitted by the quantum dot.
Types of Quantum Dots
There are two types:- epitaxial quantum dots;
- colloidal quantum dots.
Quantum dot design
Typically, a quantum dot is a semiconductor crystal in which quantum effects are realized. An electron in such a crystal feels like it is in a three-dimensional potential well and has many stationary energy levels. Accordingly, when moving from one level to another, a quantum dot can emit a photon. With all this, the transitions are easy to control by changing the dimensions of the crystal. It is also possible to transfer an electron to a high energy level and receive radiation from the transition between lower-lying levels and, as a result, we obtain luminescence. Actually, it was the observation of this phenomenon that served as the first observation of quantum dots.Now about the displays
The history of full-fledged displays began in February 2011, when Samsung Electronics presented the development of a full-color display based on QLED quantum dots. It was a 4-inch display controlled by an active matrix, i.e. Each color quantum dot pixel can be turned on and off by a thin film transistor.To create a prototype, a layer of quantum dot solution is applied to a silicon circuit board and a solvent is sprayed on. Then a rubber stamp with a comb surface is pressed into the layer of quantum dots, separated and stamped onto glass or flexible plastic. This is how stripes of quantum dots are applied to a substrate. In color displays, each pixel contains a red, green or blue subpixel. Accordingly, these colors are used with different intensities to obtain the best possible more shades.
The next step in development was the publication of an article by scientists from the Indian Institute of Science in Bangalore. Where were quantum dots described that not only luminesce? orange, but also in the range from dark green to red.
Why is LCD worse?
The main difference between a QLED display and an LCD is that the latter can cover only 20-30% of the color range. Also in QLED TVs there is no need to use a layer with light filters, since the crystals, when voltage is applied to them, always emit light with a clearly defined wavelength and, as a result, with the same color value.There was also news about the sale of a computer display based on quantum dots in China. Unfortunately, I haven’t had a chance to check it with my own eyes, unlike on TV.
P.S. It is worth noting that the scope of application of quantum dots is not limited only to LED monitors; among other things, they can be used in field effect transistors, photocells, laser diodes, the possibility of using them in medicine and quantum computing.
P.P.S. If we talk about my personal opinion, then I believe that they will not be popular for the next ten years, not because they are little known, but because the prices for these displays are sky-high, but I still want to hope that quantum the points will find their application in medicine, and will be used not only to increase profits, but also for good purposes.
A.V. Fedorov, A.V. Baranov | ||||||||||||
Ln[ K(τ ) ] | ||||||||||||
τ, ps |
Rice. 4.32. a is the logarithm of the coherent monitoring signal envelope as a function of the mutual delay between pulses for various relative contributions of Lorentzian homogeneous and Gaussian inhomogeneous broadenings (r = 2 = ! ). Solid line – purely Lorentzian homogeneous broadening with ~ 2 = 21:25 µeV; dashed line –r =1/1; dotted line –r =1/2.5; dash-dotted –r =1/14. Absolute values2 and! were chosen in such a way that the HWHM of the photoluminescent line of a single quantum dot was kept constant (21:25 μeV) in accordance with the work. b – Voigt contour of the photoluminescent line of a single quantum dot, calculated for the same parameters as in case a.
measuring device and adjustment with the Voigt circuit. This leads to additional errors. In Fig. 4.32 b the shapes of the photoluminescence lines of a single quantum dot are plotted for the same ratios2 = ! , as in Fig. 4.32 a. It can be seen that the most informative part of the spectral lines is their wings, where it is difficult to achieve good attitude signal/noise At the same time, the corresponding changes in K() are most pronounced in the region where the coherent control signal can be obtained with sufficient accuracy. Thus, the coherent control method can be used to study the effects of fluctuations of the charge environment in optical and relaxation processes.
4.3. Applications of Quantum Dots
4.3.1. Quantum dot lasers for fiber communications
The development of fiber optic telecommunications has led to the need to create efficient semiconductor lasers and optical amplifiers operating in the spectral region of minimal waveguide losses (1.25–1.65 µm). Maximum length The wavelength achieved by InGaAs/GaAs quantum well lasers is 1230 nm for devices that generate from the end, and 1260 nm for lasers with a vertical cavity. Sufficiently large threshold currents, low operating temperature and low
4. Quantum dot optics |
The temperature stability of such lasers does not always meet the requirements for high-speed telecommunications devices.
Progress in the fabrication of multilayer structures of self-organized quantum dots of A3 B5 compounds, sufficiently uniform in size and shape at large surface density, led to the creation of semiconductor lasers with quantum dots as the active medium. As a result, the spectral region of 1.0–1.7 μm has become available for lasing both for lasers of traditional design and for lasers with a vertical cavity using InGaAs quantum dots and GaAs substrates. In particular, both types of lasers can generate radiation at a wavelength of 1.3 µm with extremely low threshold currents and high output power. Recently, a broadband quantum dot laser was demonstrated, emitting at 1.5 μm with a current density of only 70 A/cm2 per layer of quantum dots at room temperature. Optical amplifiers based on quantum dot structures are of interest for high-speed signal processing at speeds above 40 Gbit/s. It is significant that developed GaAs technologies make it possible to produce fairly cheap monolithic quantum dot lasers with a vertical cavity and distributed Bragg mirrors based on AlAs/GaAs and AlOx/GaAs pairs.
It should be noted that due to the inhomogeneous broadening of electronic transitions in quantum dots, it becomes possible to expand the region of continuous tuning of the lasing wavelength. With a slight increase in threshold currents, it can reach 200 nm (1.033–1.234 µm).
Lasers using InAs quantum dots and InP substrates are also of interest because they allow generation in the longer wavelength range (1.8–2.3 μm), which is important for applications in molecular spectroscopy and remote control gas atmospheres using lidars. At the same time, generation of radiation with wavelengths of 1.9 and 2 μm from a laser with an active medium from such a heterostructure has so far been obtained only at low (77 K) temperatures. Interestingly, lasing at wavelengths of 1.6 and 1.78 μm was also demonstrated for lasers based on InAs quantum wires – one-dimensional quantum structures on a (001)InP substrate. Finally, continuous lasing in the 2-μm region was obtained at room temperature using InAsSb-based quantum dots grown on a (001)InP substrate as the active medium of the laser.
The intensive development of this direction has led to the fact that at present some types of semiconductor lasers with an active medium based on quantum dots have become commercially available.
260 A.V. Fedorov, A.V. Baranov
4.3.2. Quantum dots in biology and medicine
One of the most actively developing areas of application of semiconductor quantum dots is the use of colloidal quantum dots (semiconductor nanocrystals in organic and aqueous solutions) as luminescent labels for visualizing the structure of biological objects different types and for ultra-sensitive detection of biochemical reactions, which are extremely important in molecular and cellular biology, medical diagnostics and therapy. A luminescent label is a phosphor associated with a linker molecule that can selectively bind to a detectable biological structure (target). Labels must be soluble in water, have a high absorption coefficient, and have a high luminescence quantum yield in a narrow spectral band. The latter is especially important for recording multicolor images, when different targets in a cell are labeled with different labels. Organic dyes are usually used as phosphors for tags. Their disadvantages are low resistance to photobleaching, which does not allow long-term measurements, the need to use several light sources to excite various dyes, as well as the large width and asymmetry of luminescence bands, which complicate the analysis of multicolor images.
Recent advances in the field of nanotechnology allow us to talk about the creation of a new class of luminescent tags using semiconductor quantum dots - colloidal nanocrystals - as a phosphor.
The synthesis of nanocrystals based on compounds A2 B6 (CdSe, CdS, CdTe, ZnS) and A3 B5 (InP and GaAs) has been known for quite a long time. Back in 1993, high-temperature organometallic synthesis of CdSe quantum dots was proposed and nanocrystals with a good crystal structure and a narrow size distribution, but with a quantum yield not exceeding 10%, were obtained. A sharp increase in the quantum yield of quantum dots to 85% at room temperature was achieved when nanocrystals began to be coated with a thin (1–2 monolayer) shell of another material with a larger band gap (for example, for CdSe this is ZnS, CdS, CdO). Such structures are called core/shell quantum dots (core/shell QDs). The diameter of quantum dots (from 1.5 nm and above) can be controlled by varying the reaction time, which takes place at a temperature of about 300o C, from minutes to several hours, or simply by selecting the required amount of product through different time after the start of the reaction. As a result, it turned out to be possible to obtain a set of quantum dots of the same composition, but with different sizes. For example, the position of the luminescence band of CdSe/ZnS QDs can vary in the range from 433 to 650 nm (2.862–1.906 eV) with a band width of about 30 meV. The use of other materials makes it possible to significantly expand the spectral range of tuning of the luminescence band of nanocrystals (Fig. 4.33). Essentially
Quantum dot optics | |||||
Intensity | |||||
Wavelength, |
Rice. 4.33. Luminescence spectra of semiconductor nanocrystals of various compositions and different sizes. Solid lines correspond to CdSe nanocrystals with diameters of 1.8, 3.0, and 6.0 nm, dotted lines correspond to InP nanocrystals with diameters of 3.0 and 4.6 nm, and dashed lines correspond to InAs nanocrystals with sizes of 2.8, 3.6, 4.6, and 6.0 nm.
that nanocrystals exhibit narrower and more symmetrical luminescence bands than conventional organic dyes. This is an extremely important advantage when analyzing multicolor images. In Fig. As an example, Fig. 4.34 compares the luminescence spectra of CdSe/ZnS nanocrystals and rhodamine 6G molecules.
Intensity, rel. units
Rhodamine 6 F |
Quantum dots
Wavelength, nm
Rice. 4.34. Comparison of luminescence bands of quantum dots and rhodamine 6G molecules.
An additional advantage is that nanocrystals of the same composition usually have wide strip absorption with a high molar extinction coefficient (up to 10−6 cm−1 M−1), corresponding to transitions to high-energy states. Its position weakly depends on the size of the quantum dot. Therefore, unlike dyes, it is possible
262 A.V. Fedorov, A.V. Baranov
efficient excitation of luminescence of nanocrystals of different sizes with one laser source Sveta. However, the main advantage is that nanocrystals have excellent photostability: they do not fade for several hours or even days, while the characteristic photobleaching times of conventional phosphors are limited to a few minutes (Fig. 4.35 AlexaFluor® 488Fig. 4.35. Photoinduced degradation of luminescence of tags based on CdSe/ZnS nanocrystals and traditional molecular phosphors under the influence of mercury lamp radiation.
The surface of such quantum dots obtained as a result chemical reaction, are coated with hydrophobic molecules used in synthesis, so they are soluble only in organic solvents. Since biological entities (proteins, DNA, peptides) exist only in aqueous solutions, methods have been developed to modify the surface of nanocrystals that make them water-soluble with both positively and negatively charged surfaces. Several types of linker molecules have been proposed that make it possible to selectively link nanocrystals with the analyzed biomolecules. As an example, Fig. 4.36 shows an example of a CdSe nanocrystal coated with a ZnS shell, which is covalently bound to a protein by a mercaptoacetic acid molecule.
Most recently, luminescent tags based on semiconductor quantum dots for targets various types became commercially available.
To use quantum dots in vivo, measures must be taken to reduce their toxicity. For these purposes, it is proposed to place quantum dots in inert polymer spheres with diameters of 50–300 nm and use them as phosphors in cases where the relatively large sizes of nanospheres do not prevent their use. Use
Any substance of microscopic size is a nanoparticle, a material used by nanotechnology researchers to design and create new technologies based on the use of elements in this tiny form. We read carefully, because we will need to delve a little into the essence of the text.
Quantum dots are nanoparticles made from any semiconductor material, such as silicon, cadmium selenide, cadmium sulfide or indium arsenide, which glow a certain color when illuminated with light.
The color they glow depends on the size of the nanoparticle. By placing quanta different sizes It is possible to achieve the presence of red, green and blue in every pixel of the display screen, which makes it possible to create a full spectrum of colors in these pixels (any existing color is created by mixing these colors).
When quantum dots are illuminated with UV light, some of the electrons gain enough energy to break free from the atoms. This ability allows them to move around the nanoparticle, creating a conduction zone in which electrons can freely move through the material and conduct electricity.

When electrons descend into the outer orbit around an atom (valence band), they emit light. The color of this light depends on the energy difference between the conduction band and the valence band.
The smaller the nanoparticle, the higher the energy difference between the valence band and the conduction band, resulting in a deeper blue color. For a larger nanoparticle, the energy difference between the valence band and the conduction band is lower, which shifts the emission toward the red.
Quantum dots and displays
For LCD displays, the benefits are numerous. Let's look at the most important and interesting features that LCD screens received from quantum dots.
Higher peak brightness
One of the reasons why manufacturers are so excited about quantum dots is the ability to create screens with much higher peak brightness than using other technologies. In turn, the increased peak brightness gives much great opportunities to use HDR and Dolby Vision.
Dolby Vision is a video standard that has high dynamic range, that is, a very large difference in light between the brightest and darkest point on the screen, which makes the image more realistic and contrasty.

If you don’t know, developers are constantly trying to play the Lord God and create what he created (or who created all this around us, maybe the universe?), only to transfer it to the screen.
That is, for example, normal sky on a clear day has a brightness of approximately 20,000 nits (brightness unit), while best tvs can provide brightness of about 10 less. So, the Dolby Vision standard is still ahead of the rest, but they are still very far from the Creator :)
Accordingly, quantum dot screens are another step towards a brighter image. Perhaps someday we will be able to see an almost real sunrise and/or sunset, and maybe other unique wonders of nature, without leaving home.
Best color rendition
Another big benefit of quantum dots is improved color accuracy. Since each pixel contains CTs of red, blue and green, it gives you access to a full palette of colors, which in turn allows you to achieve an incredible number of shades of any color.
Improved battery life for mobile devices
Quantum dot screens promise not only excellent image quality, but also extremely low power consumption.
Quantum dots and Samsung QLED
TVs based on quantum dots from Samsung, or simply, are actually not entirely based on quantum dots in the correct understanding of this technology. QLED is more of a hybrid, something between quantum dots and LED screens. Why? Because these TVs still use LED lights, and in a real screen based on quantum dots, light should be created precisely by the dots.

Therefore, even if the new TVs from the South Korean giant show better than conventional LED screens, they are still not quantum dot TVs, but TVs with quantum dots instead of a light filter.
Comments:
Ivan Ivanovich |