All about space stars. Stars. Largest in the Universe
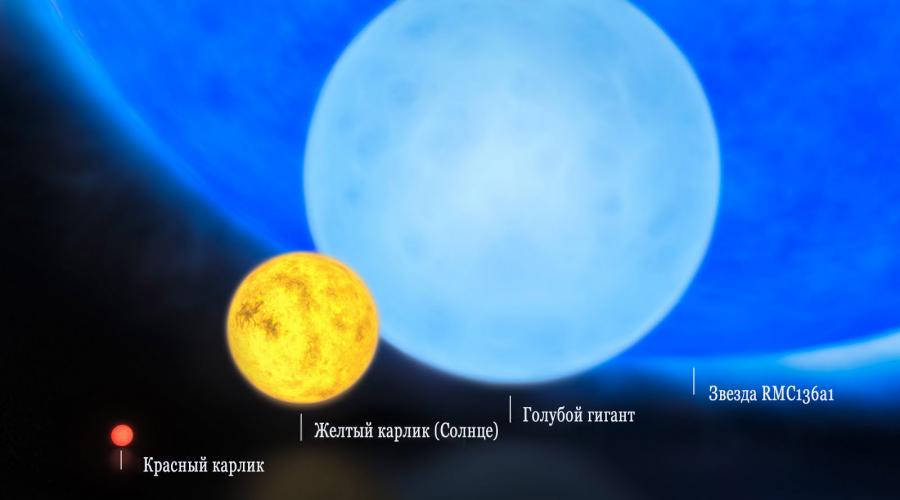
The seemingly inconspicuous UY Shield
Modern astrophysics, in terms of stars, seems to be reliving its infancy. Star observations provide more questions than answers. Therefore, when asking which star is the largest in the Universe, you need to be immediately prepared for answering questions. Are you asking about the largest star known to science, or about what limits science limits a star? As is usually the case, in both cases you will not get a clear answer. The most likely candidate for the biggest star quite equally shares the palm with its “neighbors.” How much smaller it may be than the real “king of the star” also remains open.
Comparison of the sizes of the Sun and the star UY Scuti. The Sun is an almost invisible pixel to the left of UY Scutum.
With some reservations, the supergiant UY Scuti can be called the largest star observed today. Why “with reservation” will be stated below. UY Scuti is 9,500 light-years away from us and is observed as a faint variable star, visible in a small telescope. According to astronomers, its radius exceeds 1,700 solar radii, and during the pulsation period this size can increase to as much as 2,000.
It turns out that if such a star were placed in the place of the Sun, the current orbits of a terrestrial planet would be in the depths of a supergiant, and the boundaries of its photosphere would at times abut the orbit. If we imagine our Earth as a grain of buckwheat, and the Sun as a watermelon, then the diameter of the UY Shield will be comparable to the height of the Ostankino TV tower.
To fly around such a star at the speed of light it will take as much as 7-8 hours. Let us remember that the light emitted by the Sun reaches our planet in just 8 minutes. If you fly at the same speed as it makes one revolution around the Earth in an hour and a half, then the flight around UY Scuti will last about 36 years. Now let’s imagine these scales, taking into account that the ISS flies 20 times faster than a bullet and tens of times faster than passenger airliners.
Mass and luminosity of UY Scuti
It is worth noting that such a monstrous size of the UY Shield is completely incomparable with its other parameters. This star is “only” 7-10 times more massive than the Sun. It turns out that the average density of this supergiant is almost a million times lower than the density of the air around us! For comparison, the density of the Sun is one and a half times higher than the density of water, and a grain of matter even “weighs” millions of tons. Roughly speaking, the averaged matter of such a star is similar in density to a layer of atmosphere located at an altitude of about one hundred kilometers above sea level. This layer, also called the Karman line, is the conventional boundary between the earth's atmosphere and space. It turns out that the density of the UY Shield is only slightly short of the vacuum of space!
Also UY Scutum is not the brightest. With its own luminosity of 340,000 solar, it is tens of times dimmer than the brightest stars. A good example is the star R136, which, being the most massive star known today (265 solar masses), is almost nine million times brighter than the Sun. Moreover, the star is only 36 times larger than the Sun. It turns out that R136 is 25 times brighter and about the same number of times more massive than UY Scuti, despite the fact that it is 50 times smaller than the giant.
Physical parameters of UY Shield
Overall, UY Scuti is a pulsating variable red supergiant of spectral class M4Ia. That is, on the Hertzsprung-Russell spectrum-luminosity diagram, UY Scuti is located in the upper right corner.
At the moment, the star is approaching the final stages of its evolution. Like all supergiants, it began actively burning helium and some other heavier elements. According to modern models, in a matter of millions of years, UY Scuti will successively transform into a yellow supergiant, then into a bright blue variable or Wolf-Rayet star. The final stages of its evolution will be a supernova explosion, during which the star will shed its shell, most likely leaving behind a neutron star.
Already now, UY Scuti is showing its activity in the form of semi-regular variability with an approximate pulsation period of 740 days. Considering that the star can change its radius from 1700 to 2000 solar radii, the speed of its expansion and contraction is comparable to the speed of spaceships! Its mass loss is at an impressive rate of 58 million solar masses per year (or 19 Earth masses per year). This is almost one and a half Earth masses per month. Thus, being on the main sequence millions of years ago, UY Scuti could have had a mass of 25 to 40 solar masses.
Giants among the stars
Returning to the disclaimer stated above, we note that the primacy of UY Scuti as the largest known star cannot be called unambiguous. The fact is that astronomers still cannot determine the distance to most stars with a sufficient degree of accuracy, and therefore estimate their sizes. In addition, large stars are usually very unstable (remember the pulsation of UY Scuti). Likewise, they have a rather blurred structure. They may have a fairly extensive atmosphere, opaque shells of gas and dust, disks, or a large companion star (for example, VV Cephei, see below). It is impossible to say exactly where the boundary of such stars lies. After all, the established concept of the boundary of stars as the radius of their photosphere is already extremely arbitrary.
Therefore, this number can include about a dozen stars, which include NML Cygnus, VV Cephei A, VY Canis Majoris, WOH G64 and some others. All these stars are located in the vicinity of our galaxy (including its satellites) and are in many ways similar to each other. All of them are red supergiants or hypergiants (see below for the difference between super and hyper). Each of them will turn into a supernova in a few millions, or even thousands of years. They are also similar in size, lying in the range of 1400-2000 solar.
Each of these stars has its own peculiarity. So in UY Scutum this feature is the previously mentioned variability. WOH G64 has a toroidal gas-dust envelope. Extremely interesting is the double eclipsing variable star VV Cephei. It is a close system of two stars, consisting of the red hypergiant VV Cephei A and the blue main sequence star VV Cephei B. The centra of these stars are located from each other at some 17-34 . Considering that the radius of VV Cepheus B can reach 9 AU. (1900 solar radii), the stars are located at “arm’s length” from each other. Their tandem is so close that whole pieces of the hypergiant flow at enormous speeds onto the “little neighbor”, which is almost 200 times smaller than it.
Looking for a leader
Under such conditions, estimating the size of stars is already problematic. How can we talk about the size of a star if its atmosphere flows into another star, or smoothly turns into a disk of gas and dust? This is despite the fact that the star itself consists of very rarefied gas.
Moreover, all the largest stars are extremely unstable and short-lived. Such stars can live for a few millions, or even hundreds of thousands of years. Therefore, when observing a giant star in another galaxy, you can be sure that a neutron star is now pulsating in its place or a black hole is bending space, surrounded by the remnants of a supernova explosion. Even if such a star is thousands of light years away from us, one cannot be completely sure that it still exists or remains the same giant.
Let us add to this the imperfection of modern methods for determining the distance to stars and a number of unspecified problems. It turns out that even among a dozen known largest stars, it is impossible to identify a specific leader and arrange them in order of increasing size. In this case, UY Shield was cited as the most likely candidate to lead the Big Ten. This does not mean at all that his leadership is undeniable and that, for example, NML Cygnus or VY Canis Majoris cannot be greater than her. Therefore, different sources may answer the question about the largest known star in different ways. This speaks less of their incompetence than of the fact that science cannot give unambiguous answers even to such direct questions.
Largest in the Universe
If science does not undertake to single out the largest among the discovered stars, how can we talk about which star is the largest in the Universe? Scientists estimate that the number of stars, even within the observable Universe, is ten times greater than the number of grains of sand on all the beaches of the world. Of course, even the most powerful modern telescopes can see an unimaginably smaller portion of them. It will not help in the search for a “stellar leader” that the largest stars can stand out for their luminosity. Whatever their brightness, it will fade when observing distant galaxies. Moreover, as noted earlier, the brightest stars are not the largest (for example, R136).
Let us also remember that when observing a large star in a distant galaxy, we will actually see its “ghost”. Therefore, it is not easy to find the largest star in the Universe; searching for it will simply be pointless.
Hypergiants
If the largest star is practically impossible to find, maybe it’s worth developing it theoretically? That is, to find a certain limit after which the existence of a star can no longer be a star. However, even here modern science faces a problem. The modern theoretical model of evolution and physics of stars does not explain much of what actually exists and is observed in telescopes. An example of this is hypergiants.
Astronomers have repeatedly had to raise the bar for the limit of stellar mass. This limit was first introduced in 1924 by the English astrophysicist Arthur Eddington. Having obtained a cubic dependence of the luminosity of stars on their mass. Eddington realized that a star cannot accumulate mass indefinitely. The brightness increases faster than the mass, and this will sooner or later lead to a violation of hydrostatic equilibrium. The light pressure of increasing brightness will literally blow away the outer layers of the star. The limit calculated by Eddington was 65 solar masses. Subsequently, astrophysicists refined his calculations by adding unaccounted components and using powerful computers. So the current theoretical limit for the mass of stars is 150 solar masses. Now remember that R136a1 has a mass of 265 solar masses, almost twice the theoretical limit!
R136a1 is the most massive star currently known. In addition to it, several other stars have significant masses, the number of which in our galaxy can be counted on one hand. Such stars were called hypergiants. Note that R136a1 is significantly smaller than stars that, it would seem, should be lower in class - for example, the supergiant UY Scuti. This is because it is not the largest stars that are called hypergiants, but the most massive ones. For such stars, a separate class was created on the spectrum-luminosity diagram (O), located above the class of supergiants (Ia). The exact initial mass of a hypergiant has not been established, but, as a rule, their mass exceeds 100 solar masses. None of the Big Ten's biggest stars measure up to those limits.
Theoretical dead end
Modern science cannot explain the nature of the existence of stars whose mass exceeds 150 solar masses. This raises the question of how one can determine the theoretical limit on the size of stars if the radius of a star, unlike mass, is itself a vague concept.
Let us take into account the fact that it is not known exactly what the stars of the first generation were like, and what they will be like during the further evolution of the Universe. Changes in the composition and metallicity of stars can lead to radical changes in their structure. Astrophysicists have yet to comprehend the surprises that further observations and theoretical research will present to them. It is quite possible that UY Scuti may turn out to be a real crumb against the background of a hypothetical “king star” that shines somewhere or will shine in the farthest corners of our Universe.
For many centuries, millions of human eyes, with the onset of night, direct their gaze upward - towards the mysterious lights in the sky - the stars of our Universe. Ancient people saw various figures of animals and people in clusters of stars, and each of them created its own story. Later, such clusters began to be called constellations. Today, astronomers identify 88 constellations that divide the starry sky into certain areas by which one can navigate and determine the location of stars.
Do you know the biggest star in the entire Universe?
The star VY Canis Majoris, located in the constellation Canis Major, is the largest representative of the stellar world. At the moment it is the largest star in the Universe. The star is located 5 thousand light years from the solar system. The diameter of the star is 2.9 billion km.
In our Universe, the most numerous objects accessible to the human eye are stars. They represent a source of light and energy for the entire solar system. They also create the heavy elements necessary for the origin of life. And without the stars of the Universe there would be no life, because the Sun gives its energy to almost all living beings on Earth. It warms the surface of our planet, thereby creating a warm oasis full of life among the permafrost of space. The degree of brightness of a star in the Universe is determined by its size.
But not all stars in the Universe are so huge. There are also so-called dwarf stars.
Comparative sizes of stars
Astronomers rate the size of stars on a scale according to which the brighter the star, the lower its number. Each subsequent number corresponds to a star ten times less bright than the previous one. The brightest star in the night sky in the Universe is Sirius. Its apparent magnitude is -1.46, meaning it is 15 times brighter than a star with magnitude zero.
Stars whose magnitude is 8 or more cannot be seen with the naked eye. Stars are also classified into spectral classes by color, indicating their temperature. There are the following classes of stars in the Universe: O, B, A, F, G, K, and M. Class O corresponds to the hottest stars in the Universe - blue. The coolest stars belong to class M, their color is red.
Spectral classes of stars in the Universe
Class O - 30,000-60,000K blue
Class B - 10,000-30,000K white-blue
Class A - 7500-10,000K white
Class F - 6000-7500K yellow-white
Class G - 5000-6000K yellow
Class K - 3500-5000K orange
Class M - 2000-3500K red
Contrary to popular belief, it is worth noting that the stars of the Universe do not actually twinkle. This is just an optical illusion - the result of atmospheric interference. A similar effect can be observed on a hot summer day, looking at hot asphalt or concrete. Hot air rises and it feels like you're looking through shaking glass. The same process causes the illusion of starry twinkling. The closer a star is to Earth, the more it will “twinkle” because its light passes through denser layers of the atmosphere.
Nuclear Hearth of the Universe Stars
A star in the Universe is a giant nuclear center. The nuclear reaction inside it converts hydrogen into helium, thanks to the process of fusion, which is how the star acquires its energy. Hydrogen nuclei with one proton combine to form helium atoms with two protons. The nucleus of an ordinary hydrogen atom has only one proton. Two isotopes of hydrogen also contain one proton, but also have neutrons. Deuterium has one neutron, while tritium has two. Deep inside the star, a deuterium atom combines with a tritium atom to form a helium atom and a free neutron. As a result of this long process, enormous amounts of energy are released.
For main sequence stars, the main source of energy is nuclear reactions involving hydrogen: the proton-proton cycle, characteristic of stars with masses around the Sun, and the CNO cycle, which occurs only in massive stars and only if they contain carbon. At later stages of a star’s life, nuclear reactions can occur with heavier elements, up to iron.
When a star's hydrogen supply is depleted, it begins to convert helium into oxygen and carbon. If the star is massive enough, the conversion process will continue until carbon and oxygen form neon, sodium, magnesium, sulfur and silicon. Eventually, these elements are converted into calcium, iron, nickel, chromium and copper until the core is composed entirely of metal. As soon as this happens, the nuclear reaction will stop because the melting point of iron is too high. The internal gravitational pressure becomes higher than the external pressure of the nuclear reaction and, eventually, the star collapses. The further development of events depends on the initial mass of the star.
Types of stars in the Universe
The main sequence is the period of existence of stars in the Universe, during which a nuclear reaction takes place inside it, which is the longest period of a star’s life. Our Sun is currently in this period. During this time, the star undergoes slight fluctuations in brightness and temperature. The duration of this period depends on the mass of the star. In large massive stars it is shorter, and in small ones it is longer. Very large stars have internal fuel that lasts for several hundred thousand years, while small stars like the Sun will shine for billions of years. The largest stars turn into blue giants during the main sequence.
Red giant
White dwarf
Brown dwarf
Cepheid
Double stars
Many stars in the Universe are part of large star systems. Binary stars are a system of two stars gravitationally bound to each other. They rotate in closed orbits around one center of mass. It has been proven that half of all the stars in our galaxy have a pair. Visually, paired stars look like two separate stars. They can be determined by the shift of spectrum lines (Doppler effect). In eclipsing binary systems, stars periodically eclipse each other because their orbits are located at a small angle to the line of sight.
Life cycle of stars in the Universe
A star in the Universe begins its life as a cloud of dust and gas called a nebula. The gravity of a nearby star or the blast wave from a supernova can cause the nebula to shrink. Elements of the gas cloud coalesce into a dense region called a protostar. As a result of subsequent compression, the protostar heats up. Eventually, it reaches critical mass and the nuclear process begins; Gradually the star goes through all the phases of its existence. The first (nuclear) stage of a star’s life is the longest and most stable. The lifespan of a star depends on its size. Large stars use up their vital fuel faster. Their life cycle can last no more than several hundred thousand years. But small stars live for many billions of years, as they spend their energy more slowly.
Evolution of a star
But, be that as it may, sooner or later, the stellar fuel runs out, and then the small star turns into a red giant, and the large star into a red supergiant. This phase will last until the fuel is completely used up. At this critical moment, the internal pressure of the nuclear reaction will weaken and can no longer balance the force of gravity, and as a result, the star will collapse. Small stars in the universe then typically develop into a planetary nebula with a bright, shining core called a white dwarf. Over time, it cools down, turning into a dark clot of matter - a black dwarf.
For big stars, things happen a little differently. During the collapse, they release incredible amounts of energy, and a powerful explosion gives birth to a supernova. If its magnitude is 1.4 solar magnitudes, then, unfortunately, the core will not be able to maintain its existence and, after the next collapse, the supernova will become neutron. The internal matter of the star will compress to such an extent that the atoms form a dense shell consisting of neutrons. If the stellar magnitude is three times the solar magnitude, then the collapse will simply destroy it, erase it from the face of the Universe. All that will remain of it is an area of strong gravity, nicknamed a black hole.
The nebula left behind by a star in the Universe can expand over millions of years. In the end, it will be affected by the gravity of a neighboring star or the blast wave of a supernova and everything will happen again. This process will occur throughout the Universe - an endless cycle of life, death and rebirth. The result of this stellar evolution is the formation of heavy elements necessary for life. Our solar system originated from the second or third generation of the nebula, and due to this, there are heavy elements on Earth and other planets. This means that there are pieces of stars in each of us. All the atoms of our body were born in an atomic source or as a result of a destructive supernova explosion.
List of the brightest stars visible from Earth
Sirius
The star Sirius or Alpha Canis Major is the brightest star in the constellation Canis Major. With an apparent magnitude of -1.46, Sirius is the brightest star in the sky (other than the Sun). Its absolute magnitude is 1.45, and it is located at a distance of 8.6 light years.
Sirius has a spectral class of A1Vm, a surface temperature of 9940° Kelvin and a luminosity 25 times greater than that of the Sun. The mass of Sirius is 2.02 solar masses, the diameter is 1.7 times greater than that of the Sun.
The image above shows an uncropped photograph of the star Sirius (North is up) taken with the Takahashi E-180 astrograph.
Sirius is actually a binary star system, consisting of a main sequence star designated Sirius A (spectral class A1Vm) and a faint white dwarf (spectral class DA2) designated Sirius B. The distance between Sirius A and its companion varies between 8.1 and 31.5 astronomical units. The star Sirius is so bright due to its high intrinsic luminosity and proximity to Earth. Located 8.6 light years (2.6 parsecs) away, the Sirius system is one of Earth's closest neighbors. For the Northern Hemisphere, it is observed between 30 and 73 degrees latitude. Sirius is the closest star to us that can be seen with the naked eye. Although Sirius is 25 times brighter than the Sun, it has a significantly lower luminosity than other bright stars such as Canopus, Deneb and Rigel.
The Sirius system is about 200-300 million years old. The system originally consisted of two bright bluish stars. The more massive Sirius B, consuming its resources, became a red giant before ejecting its outer layers and becoming a white dwarf about 120 million years ago. In conversation, Sirius is known as the "Dog Star", reflecting its affiliation with the constellation Canis Major. The sunrise of Sirius marked the flooding of the Nile in Ancient Egypt. The name Sirius comes from the ancient Greek “luminous” or “incandescent”.
Canopus
The star Canopus or Alpha Carinae is the brightest star in the constellation Carina. With an apparent magnitude of -0.72, Canopus is the second brightest star in the sky. Its absolute magnitude is -5.53, and it is distant from us at a distance of 310 light years.
Canopus has a spectral class of A9II, a surface temperature of 7350° Kelvin and a luminosity of 13,600 times that of the Sun. The star Canopus has a mass of 8.5 solar masses and a diameter 65 times that of the Sun.
The image above shows an uncropped photograph of the star Canopus (North is Up) taken with the Takahashi E-180 Astrograph.
Canopus is a supergiant of spectral class F and appears white when viewed with the naked eye. With a luminosity of 13,600 times that of the Sun, Canopus is essentially the brightest star, up to 700 light-years from the Solar System. If Canopus were located at a distance of 1 astronomical unit (the distance from the Earth to the Sun), it would have an apparent magnitude of -37 (for the Sun - 26.72
The diameter of the star Canopus is 0.6 astronomical units, or 65 times that of the Sun. If Canopus were located at the center of the solar system, its outer edges would extend three-quarters of the way to Mercury. The Earth had to be removed at a distance three times the orbit of Pluto in order for Canopus to appear in the sky just like our Sun.
Canopus is a strong source of X-rays, which are likely produced by its corona, which is heated to 15 million degrees Kelvin. It is a member of the Scorpius-Centauri group of stars that share a common origin.
Arcturus
The star Arcturus or Alpha Bootes is the brightest star in the constellation Bootes. With an apparent magnitude of -0.04, Arcturus is the fourth brightest star in the sky. Its absolute magnitude is -0.3 and it is distant from us at a distance of 34 light years.
The star Arcturus has a spectral class of K1.5IIIp, a surface temperature of 4300° Kelvin and a luminosity 210 times greater than that of the Sun. Its mass is 1.1 solar masses, and its diameter is 26 times the diameter of the Sun.
The image above shows an uncropped photograph of the star Arcturus (North is Up) taken with the Takahashi E-180 Astrograph.
Arcturus is visible in both hemispheres of the sky as it is located less than 20 degrees north of the celestial equator. The star reaches its zenith at midnight on April 30. There is an easy way to find the star Arcturus. You just need to follow the handle of the Big Dipper's bucket. Continuing in this direction you can find Spica. Arcturus is a star in the local interstellar cloud.
Arcturus is an orange giant of spectral class K1.5IIIp. The "P" stands for "exceptional emission," indicating that the spectrum of light coming from the star is unusual and full of emission lines. This phenomenon is not very common among red giants, but is typical for the star Arcturus. The star is at least 110 times visually brighter than the Sun, and that doesn't take into account the fact that most of the star's light is in the infrared. The total (bolometric) power is 180 times greater than that of the Sun.
Arcturus is notable for its high speed of its own movement. It is greater than the speed of any first magnitude star in the vicinity except Alpha Centauri. The star Arcturus is moving rapidly (122 km/s) compared to the Solar System and is currently at almost its closest point to the Sun. It will take another 4,000 years for the star to move a few hundredths of a light year closer to Earth than it is today. Arcturus is considered an old star and moves with a group of 52 other similar stars. This movement is known as the Arcturus Stream. Its mass is quite difficult to determine, but it is estimated to be 1.1 solar masses.
Vega
The star Vega or Alpha Lyrae is the brightest star in the constellation Lyra. With an apparent magnitude of 0.03, Vega is the fifth brightest star in the sky. Its absolute magnitude is 0.6, its distance from Earth is 25 light years.
Vega has a spectral class of A0Va, a surface temperature of 9600° Kelvin, and its luminosity is 37 times greater than that of the Sun. The mass of the star is 2.1 solar masses, the diameter is 2.3 times greater than that of the Sun.
The image above shows an uncropped photograph of the star Vega (North Up) taken with the Takahashi E-180 Astrograph.
Vega is a relatively nearby star, located 25 light years from Earth. Together with Arcturus and Sirius, it is one of the brightest stars in the vicinity of the Sun. Vega is one of the peaks of the Summer Triangle along with Deneb and Altair. Since it is located high in the sky, it is clearly visible throughout the summer months.
Vega has a spectral class of A0Va, making it a white main sequence star with a bluish tint. Currently, its age is estimated at 455 million years. Vega's age is only one-tenth the age of the Sun, but given that it is 2.1 times more massive, its expected lifespan would also be only a tenth of the Sun's. Both stars have now reached the midpoint of their lives. Vega has an unusually low abundance of elements with an atomic number greater than helium.
It is also assumed that Vega is a variable star that varies slightly in magnitude on a periodic basis. It rotates quite quickly, with a speed at the equator reaching 274 km/s. This causes the equator to bulge outward under the influence of centrifugal force and, as a result, a temperature change occurs throughout the star's photosphere, reaching a maximum at the poles. From Earth, Vega is observed from one of these poles.
Based on the observed excess of infrared emission, Vega most likely has a circumstellar dusty disk. This dust, which is the result of collisions between objects, forms an orbital debris disk, similar to the Kuiper belt in the solar system. Stars that exhibit an excess of infrared radiation are called Vega stars. The instability of Vega's disk also suggests the presence of at least one planet the size of Jupiter.
Vega was a north pole star until 12,000 BC. and will remain so after 13700 AD. Vega was the first star (after the Sun) to be photographed and the first whose spectrum was recorded. It was also one of the first stars whose distance was estimated by parallax measurements.
Chapel
Star Capella or Alpha Aurigae is the brightest star in the constellation Auriga. With an apparent magnitude of 0.08, Capella is the sixth brightest star in the sky. Its absolute magnitude is -0.5, and its distance from Earth is 41 light years.
Capella has a spectral class of G6III + G2III, a surface temperature of 4940° Kelvin, and its luminosity is 79 times greater than that of the Sun. The star's mass is 2.69 times that of the Sun, and its diameter is 12 times that of the Sun.
The image above shows an uncropped photograph of the star Capella (North is up) taken with the Takahashi E-180 astrograph.
Although Capella appears to be a single star to the naked eye, it is actually formed by two binary pairs. The first pair consists of two bright giant G-type stars with a radius 10 times greater than that of the Sun and closely related. These stars are believed to be on the path to becoming red giants.
The first star has a surface temperature of about 4900 K, a radius 12 times that of the Sun, a mass of 2.7 solar masses, and a luminosity 79 times that of the Sun. The second star has a surface temperature of about 5700K, a radius equal to 9 solar radii, a mass of 2.6 solar masses and a luminosity 78 times greater than the Sun. Although the main star is brighter when viewed at all wavelengths, it appears fainter when observed in visible light, with an apparent magnitude of approximately 0.91, compared to an apparent apparent magnitude of 0.76.
The second binary pair consists of two faint, small and relatively cool red dwarfs. The pair is located at a distance of 10,000 astronomical units (100 million km) and has an orbital period of about 104 days. Apparently, the stars have been main sequence stars of spectral class A throughout their entire lives, but at the moment they are expanding, cooling and becoming red giants. This process will take them another few million years.
Rigel
The star Rigel or Beta Orionis is the brightest star in the constellation Orion. With an apparent magnitude of 0.12, Rigel is the seventh brightest star in the sky. Its absolute magnitude is -7 and is located at a distance of ~870 light years from us.
Rigel has a spectral class of B8Iae, a surface temperature of 11,000° Kelvin, and its luminosity is 66,000 times greater than that of the Sun. The star has a mass 17 solar masses and a diameter 78 times that of the Sun.
The image above shows an uncropped photograph of the star Rigel (North is Up) taken with the Takahashi E-180 astrograph.
Rigel is the brightest star in our local region of the Milky Way. The star is so bright that when viewed from a distance of one astronomical unit (the distance from the Earth to the Sun), it will shine as an extremely bright ball with an angular diameter of 35° and an apparent magnitude of -38. The power flow at this distance will be the same as from a welding arc at a distance of several millimeters. Any object located so close will evaporate under the influence of a strong stellar wind.
Rigel is currently passing through the nebula region. Consequently, the star illuminates several dust clouds located nearby. The most prominent of these is IC 2118 (the Witch's Head Nebula). Rigel is also associated with the Orion Nebula (M42), which is more or less in visual line with the star, although it is located almost twice as far from Earth.
Rigel is a famous binary star that was first observed by Vasily Yakovlevich Struve in 1831. Although Rigel B has a relatively faint magnitude, its proximity to Rigel A, which is 500 times brighter, makes it a target for amateur astronomers. According to calculations, Rigel B is removed from Rigel A at a distance of 2200 astronomical units. Due to such a colossal distance between them, there is no sign of orbital motion, although they have the same proper motion.
Rigel B is itself a spectroscopic binary system, consisting of two main sequence stars orbiting a common center of gravity every 9.8 days. Both stars belong to the spectral type B9V.
Rigel is a variable star, which is not common among supergiants, with a magnitude range of 0.03-0.3, changing every 22-25 days.
Procyon
The star Procyon or Alpha Canis Minor is the brightest star in the constellation Canis Minor. With an apparent magnitude of 0.38, Procyon is the eighth brightest star in the night sky. Its absolute magnitude is 2.6, and its distance to Earth is 11.4 light years.
Procyon has a spectral class of F5IV-V, a surface temperature of 6650° Kelvin and a luminosity 6.9 times greater than that of the Sun. The mass of the star is 1.4 times the mass of the Sun, and its diameter is 2 times.
The image above shows an uncropped photograph of the star Procyon (North is Up) taken with the Takahashi E-180 Astrograph.
To the naked eye, Procyon appears as a single star. In fact, Procyon is a binary star system consisting of a main sequence white dwarf (spectral class F5 IV-V) called Procyon A and a faint white dwarf (spectral class DA) called Procyon B. Procyon does not look so bright due to its luminosity, but because of its proximity to the Sun. The system is located 11.46 light years (3.51 parsecs) away and is one of our closest neighbors.
Procyon A's surface temperature is estimated to be 6530° Kelvin, giving it a white hue. The mass of Procyon A is 1.4 solar masses, its radius is equal to two radii of the Sun, and its luminosity is 6.9 times greater than that of the Sun. Procyon A is quite bright for its class, implying the complete conversion of hydrogen to helium in its core. Eventually, the star will begin to expand and increase in volume by 80 to 150 times. This should happen within 10 to 100 million years.
Like Sirius B, Procyon B is a white dwarf that was isolated as a separate entity long before it was observed. Its existence was first predicted by Friedrich Bessel in 1844. Although its orbital characteristics were calculated by Arthur Overs in 1862, Procyon B was not visually confirmed until 1896, when John Martin Scheberle observed it at its predicted coordinates using the 36-inch refractor at Lick Observatory.
With a mass of 0.6 solar masses, Procyon B is significantly less massive than Sirius B. However, Procyon B's structural feature is such that it is larger than its more famous neighbor, with an estimated radius of 8600 km, compared to 5800 km for Sirius B. Surface Temperatures star Procyon B is 7740° Kelvin, which is also much colder than Sirius B. This indicates its lower mass and greater age. Procyon B's progenitor star had a mass of about 2.5 solar masses, and it came to the end of its life about 1.7 billion years ago. For this reason, Procyon A is believed to be 2 billion years old.
The star Procyon forms one of the three vertices of the Winter Triangle, along with Sirius and Betelgeuse.
Betelgeuse
The star Betelgeuse or Alpha Orionis is the second brightest star in the constellation Orion. With an apparent magnitude of 0.5, Betelgeuse is the ninth brightest star in the night sky. Its absolute magnitude is -5.14, and its distance to Earth is 530 light years.
Betelgeuse has a spectral class of M2Iab, a surface temperature of 3500° Kelvin and a luminosity 140,000 times that of the Sun. The star has a mass equal to 18 solar masses and a diameter equal to 1180 times the diameter of the Sun.
The image above shows an uncropped photograph of the star Betelgeuse (North is Up) taken with the Takahashi E-180 Astrograph.
The red supergiant Betelgeuse is one of the largest and most luminous stars known. If it were located at the center of our solar system, its surface would engulf the entire inner solar system (Mercury, Venus, Earth and Mars), extend beyond the asteroid belt and possibly reach Jupiter. However, due to the fact that the distance between the star and the Earth has changed over the last century from 180 to 1300 light years, it is quite difficult to calculate its diameter and luminosity. Betelgeuse is currently believed to be located 640 light-years from Earth, giving it an average absolute magnitude of about -6.05.
In 1920, Alpha Orionis became the first star (after the Sun) to have its angular diameter measured. Since then, researchers have used a number of telescopes to measure this giant star, each with different technical parameters, often yielding conflicting results. The star's current apparent diameter ranges from 0.043 to 0.056 seconds. This is a real moving target, as the star Betelgeuse periodically changes shape. In addition, Betelgeuse has a complex, asymmetrical envelope caused by the enormous loss of mass due to huge jets of gas escaping from the surface. There is even evidence that Betelgeuse has a stellar companion orbiting in its gaseous envelope, contributing to the star's eccentric behavior.
Betelgeuse is believed to be only 10 million years old, but it evolved quickly due to its high mass. The star appears to be an escapee from the Orion OB1 star cluster, which includes O and B type stars in Orion's belt (Alnitak, Alnilam and Mintaka). Betelgeuse is currently in a late stage of evolution and is expected to explode as a Type II supernova in the next millions of years.
Having a distinct reddish hue, it is a semi-regular variable star with an apparent magnitude varying between 0.2 and 1.2. The star is the upper right corner of the Winter Triangle, along with Sirius and Procyon.
Betelgeuse is easy to spot in the night sky as it appears so close to the famous Belt of Orion. In the northern hemisphere, it can be seen growing in the east just after sunset in January. By mid-March, the star appears in the south in the evening sky and is visible in almost every populated region of the globe. In major cities in the southern hemisphere (such as Sydney, Buenos Aires and Cape Town), the star rises almost 49° above the horizon.
Altair
The star Altair or Alpha Aquila is the brightest star in the constellation Aquila. With an apparent magnitude of 0.77, Altair is the 12th brightest star in the night sky. Its absolute magnitude is 2.3, and its distance to Earth is 18 light years.
Altair has a spectral class of A7Vn, a surface temperature of 7500° Kelvin and a luminosity 10.6 times greater than that of the Sun. Its mass is equal to 1.79 solar masses, and its diameter is 1.9 times larger than that of the Sun.
The image above shows an uncropped photograph of the star Altair (North Up) taken with the Takahashi E-180 astrograph.
Located 18 light years (5.13 parsecs) away, Altair is one of the closest stars visible to the naked eye. Along with Beta Orla and Tarazed, the star forms the well-known lineage of stars sometimes called the Aquila family. Altair makes up one of the vertices of the Summer Triangle along with Deneb and Vega.
The star Altair is an A-type main sequence star. It has an extremely high rotation speed, reaching 210 kilometers per second at the equator. Thus, one period is about 9 hours. By comparison, the Sun takes just over 25 days to complete one revolution around the equator. This rapid rotation causes Altair to be slightly flattened. Its equatorial diameter is 20 percent larger than its polar diameter.
Aldebaran
The star Aldebaran or Alpha Tauri is the brightest star in the constellation Taurus. With an apparent magnitude of 0.85, Aldebaran is the 14th brightest star in the night sky. Its absolute magnitude is -0.3, and its distance from Earth is 65 light years.
Aldebaran has a spectral class of K5III, a surface temperature of 4010° Kelvin and a luminosity 425 times greater than that of the Sun. The star Aldebaran has a mass of 1.7 solar masses and a diameter that is 44.2 times the diameter of the Sun.
The image above shows an uncropped photograph of the star Aldebaran (North is Up) taken with the Takahashi E-180 Astrograph.
Aldebaran is an orange giant that moved with the main sequence line of the Hertzsprung-Russell diagram. It ran out of hydrogen fuel in its core and the hydrogen fusion process stopped. Although it is not yet high enough for helium fusion, the temperature of the star's core increased significantly due to gravitational pressure, and the star expanded to 44.2 times the diameter of the Sun, reaching a value of 61 million kilometers. The Hipparcos satellite measured the distance to the star to be 65 light years (20.0 parsecs). Aldebaran is a slightly variable LB type star. Its fluctuations in apparent magnitude are approximately 0.2.
Aldebaran is one of the simplest stars to find in the night sky, partly due to its brightness and partly due to its spatial location in relation to one of the most prominent asterisms in the sky. If you follow the three stars of Orion's belt from left to right (in the northern hemisphere) or from right to left (in the southern hemisphere), the first bright star you will find as you continue along this line is Aldebaran.
Aldebaran is the brightest member of the Hyades group of open star clusters, which makes up the "bull's head" in the constellation Taurus. However, Aldebaran simply happens to be in the direct line of sight between Earth and the Hyades. The star cluster is actually located twice as far away, at a distance of 150 light years.
The name Aldebaran comes from Arabic and literally translates to "follower", apparently due to the fact that this bright star appears to follow the Pleiades or the Seven Sisters star cluster in the night sky.
Antares
The star Antares or Alpha Scorpius is the brightest star in the constellation Scorpius. With an apparent stellar magnitude of 0.96, Antares is the 16th brightest star in the sky. Its absolute magnitude is -5.28, and its distance from Earth is 604 light years.
Antares has a spectral class of M1.5Iab, a surface temperature of 3500° Kelvin and a luminosity 65,000 times that of the Sun. The mass of the star is 15.5 solar masses, and its diameter is 800 times greater than that of the Sun.
The image above shows an uncropped photograph of the star Antares (North is Up) taken with the Takahashi E-180 astrograph.
Antares is a supergiant. If it is placed in the center of the solar system, then its outer surface will be between the orbits of Mars and Jupiter. Based on parallax measurements, Antares is 550 light years (170 parsecs) from Earth. Antares has a visual luminosity 10,000 times that of the Sun, but since the star emits a significant amount of energy in the infrared, its bolometric luminosity is 65,000 times greater than that of the Sun. Antares is also an irregular variable star (type LC), whose apparent magnitude ranges from 0.88 to 1.16.
Antares is at opposition to the Sun around May 31st of each year. At this time, the star is visible throughout the night. For about two to three weeks before and after November 30, Antares is not visible in the night sky as it is lost in the brilliance of the Sun. Along with Aldebaran, Spica and Regulus, Onya is one of the four brightest stars located near the ecliptic.
Antares has a secondary companion star, Antares B, whose angular separation changed from 3.3 arcseconds in 1854 to 2.86 arcseconds in 1990. The star is usually difficult to see due to the glare coming from Antares A.
Spica
The star Spica or Alpha Virgo is the brightest star in the constellation Virgo. With an apparent magnitude of 0.98, Spica is the 15th brightest star in the night sky. Its absolute magnitude is -3.2, and its distance from Earth is 262 light years.
Spica has a spectral class of B1V, a surface temperature of 22,400° Kelvin and a luminosity 12,100 times that of the Sun. Its mass reaches 10.3 solar masses, and its diameter is 7.4 times the diameter of the Sun.
The image above shows an uncropped photograph of the star Spica (North Up) taken with the Takahashi E-180 astrograph.
Spica is a close binary star whose components orbit a common center of mass every four days. They are located close enough to each other that they cannot be detected in a telescope as two separate stars. Changes in the orbital motion of this pair results in a Doppler shift in the absorption lines of their respective spectra, making them a spectral binary pair. The orbital parameters for this system were first derived using spectroscopic measurements.
The main star has a spectral class of B1 III-IV. The luminosity class does not match the spectrum of the star, which is between a subgiant and a giant star, and it is no longer a main sequence B-type star. It is a massive star, with a mass 10 times the mass of the Sun and a radius seven times greater. The total luminosity of this star is 12,100 times greater than that of the Sun and eight times greater than that of its companion. The main star of this pair is one of the closest stars to the Sun, which has enough mass to end its life in a Type II supernova explosion.
The main star is classified as a Beta Cephei variable star, which changes in brightness by a value of 0.1738 every day. The spectrum shows a variation in radial velocity with the same period, indicating that the star's surface is pulsating regularly. This star is spinning fast. The rotation speed along the equator is 199 km/s.
The secondary star of this system is one of the few stars that exhibits the Struve-Sahade effect. This is an anomalous change in the strength of spectral lines during orbit, where the lines become weaker as the star moves away from the observer. This star is smaller than the main one. Its mass is seven times that of the Sun, and the radius of the star is 3.6 solar radii. The star has a spectral class of B2 V, making it a main sequence star.
Spica is an ellipsoidal variable where stars are distorted by gravitational interactions. This effect causes a change in the apparent magnitude of the stellar system by a value equal to 0.03 over a time interval that corresponds to the orbital period. This slight decrease in magnitude is barely noticeable visually. The rotation rates of both stars are faster than their orbital periods. This lack of synchronization and the highly elliptical nature of their orbit may indicate that they are a young star system. Over time, the pair's mutual tidal interaction can lead to rotational synchronization and orbital cyclization.
Pollux
The star Pollux or Beta Gemini is the brightest star in the constellation Gemini. With an apparent magnitude of 1.14, Pollux is the 17th brightest star in the sky. Its absolute magnitude is 0.7, and its distance to Earth is 40 light years.
Pollux has a spectral class of K0IIIb, a surface temperature of 4865° Kelvin and a luminosity 32 times greater than that of the Sun. Its mass is equal to 1.86 solar masses, and its diameter is 8 times larger than that of the Sun.
The image above shows an uncropped photograph of the star Pollux (North is Up) taken with the Takahashi E-180 Astrograph.
The twin stars Castor and Pollux are best visible during northern spring evenings. Unlike real-life twins, Castor and Pollux have little in common. Castor is a white quadruple star composed of fairly similar white components (spectral class A), while Pollux is an orange-colored cool giant (spectral class K0IIIb).
Close conjugation with Castor gives Pollux a brighter color. The star, located 34 light years away, has a total luminosity 46 times greater than the Sun. With its cold temperature (4770° Kelvin) and a diameter that is 10 times that of the Sun, Pollux is smaller than most of its cool giant cousins and is only a quarter the diameter of Aldebaran. In its deep core, the process of fusion of hydrogen into helium occurs, which is typical for most red giants. The star emits X-rays and appears to have a magnetized corona.
In 2006, an exoplanet was discovered orbiting Pollux, making it the brightest star in the sky with a known exoplanet. With a mass that is at least 2.9 times that of Jupiter, the planet floats in a circular orbit at a distance of 1.69 astronomical units, with a rotation period of 590 days (1.6 years).
Fomalhaut
The star Fomalhaut or Alpha Pisces Southernis is the brightest star in the constellation Pisces Southernes. With an apparent magnitude of 1.16, Fomalhaut is the 18th brightest star in the sky. Its absolute magnitude is 2.0, and it is located at a distance of 22 light years.
Fomalhaut has a spectral class of A3Va, a surface temperature of 8750° Kelvin and a luminosity 17.9 times that of the Sun.
The image above shows an uncropped photograph of the star Fomalhaut (North is Up) taken with the Takahashi E-180 Astrograph.
Fomalhaut is a relatively young star, about 300 million years old, with a potential lifespan of up to a billion years. The star is metal deficient compared to the Sun, meaning it is made up of a smaller percentage of elements other than hydrogen and helium. A star's metallicity is determined by measuring the abundance of iron in the photosphere relative to hydrogen. In 1997, spectroscopic studies showed a value equal to 93% of the volume of iron in the Sun, but more recent studies have shown that the value may actually be half that.
Fomalhaut is one of 16 stars belonging to the Castor moving group of stars. This is an association of stars that shares the common motion of stars in space and therefore can be physically connected. Other members of this group are Castor and Vega. Scientists estimate that this moving group is about 200 million years old. The neighboring star TW Pisces Southernes, which is also a member of this group, may form a physical pair with Fomalhaut.
Fomalhaut is surrounded by a toroidal-shaped dust disk of debris with a very sharp inner edge at a radial distance of 133 AU. The dust is distributed in a belt approximately 25 AU wide and is sometimes referred to as the "Kuiper Fomalhaut Belt". The dusty disk of Fomalhaut is believed to be protoplanetary and emits infrared radiation. Measurements of Fomalhaut's rotation indicate that the disk is in the equatorial plane of the star, as predicted by the theory of star and planet formation.
Fomalhaut is of particular importance in exosolar research as it is the center of the first star system with an exoplanet (Fomalhaut b) seen at visible wavelengths. The mass of the planet is approximately no more than three times the mass of Jupiter and no less than the mass of Neptune.
Deneb
The star Deneb or Alpha Cygnus is the brightest star in the constellation Cygnus. With an apparent magnitude of 1.25, Deneb is the 19th brightest star in the sky. Its absolute magnitude is -7.2, and its distance from Earth is 1550 light years.
Deneb has a spectral class of A2Ia, a surface temperature of 8525° Kelvin and a luminosity 54,000 times that of the Sun. Its mass is equal to 20 solar masses, and its diameter is equal to 110 solar diameters.
The image above shows an uncropped photograph of the star Deneb (North is Up) taken with the Takahashi E-180 astrograph.
Deneb, together with Altair and Vega, form the vertices of the Summer Triangle. With an absolute magnitude of 7.2, Deneb is one of the brightest stars we know. Its luminosity is estimated to be 60,000 times that of the Sun. Its exact distance to Earth is unknown, making determinations of many of Deneb's other properties also imprecise. However, the veil of uncertainty over this star was lifted by research in 2007. According to the results, the most likely distance at which the star is located is about 1,550 light years. The calculation error allows a distance of 1340 to 1840 light years. Denebae is the most distant known first magnitude star.
Based on its temperature and luminosity, as well as direct measurements of its tiny angular diameter (just 0.002 arcseconds), Deneb appears to have a diameter that is 110 times larger than that of the Sun. If placed at the center of our solar system, Deneb would occupy half the path of Earth's orbit. Alpha Cygni is one of the largest white stars we know.
The supergiant's blue-white color and high mass and temperature mean the star will have a very short lifespan and will likely go supernova within a few million years. In its core, the process of hydrogen fusion has already stopped. Deneb is currently likely expanding into a red supergiant like Mu Cephei. While it is there, the star will pass through spectral classes F, G, K and M.
Deneb's solar wind causes it to lose mass at a rate of 0.8 millionths of the sun's mass per year, which is 100,000 times the flux from the Sun. It is the prototype of a class of variable stars known as alpha Cygni variables. Its surface is subject to non-radial vibrations, which cause changes in its brightness and spectral class.
Regulus
The star Regulus or Alpha Leo is the brightest star in the constellation Leo. With an apparent magnitude of 1.35, Regulus is the 21st brightest star in the sky. Its absolute magnitude is -0.3, and the distance to Earth is 69 light years.
Regulus has a spectral class of B7Vn, a surface temperature of 10300° Kelvin and a luminosity 150 times greater than that of the Sun. The mass of the star is 3.5 times the mass of the Sun, and its diameter is 3.2 times the diameter of the Sun.
The image above shows an uncropped photograph of the star Regulus (North Up) taken with the Takahashi E-180 Astrograph.
Regulus is a multiple star system consisting of four stars. Regulus A is a double star system consisting of a bluish-white main sequence star (spectral class B7V), in whose orbit a white dwarf is believed to have a mass of 0.3 solar masses. It takes the two stars about 40 days to complete one orbit around their common center of mass.
The main star, Regulus A, is a young star with a mass of about 3.5 solar masses and is several hundred million years old. The star rotates quite quickly. Its period is only 15.9 hours, which leads to a distortion of the star's shape and to the so-called gravitational eclipse: the photosphere at the poles of this star is much hotter and five times brighter per unit surface area than at the equatorial region. If it rotated 16% faster, then the star's gravity would be weaker than the centrifugal force and the star would tear itself apart.
Given the highly distorted shape of the primary star, the relative orbital motion of the binary pair may differ dramatically from the pure Kepler two bodies due to constant perturbations affecting their orbital period. In other words, Kepler's third law, which is defined for two point masses, does not apply to this binary pair because the shape of the main star is too distorted.
At a distance of about 4200 astronomical units from Regulus A there is a double star system, which is shared by a common proper rotation. Designated Regulus B (spectral type K2V) and Regulus C (spectral type M4V), the pair have an orbital period of 2000 years and are separated by about 100 astronomical units.
The light emanating from this pair of stars dominates the binary pair Regulus A. Regulus B, when viewed in isolation, is a binocular object with an apparent magnitude 8.1 star, while its stellar companion Regulus is magnitude 13.5. Regulus A is a spectroscopic double star: the secondary star of this pair has not yet been under direct observation, since it is much fainter than the main one. Pair B and C are located at an angular distance of 177 arcseconds from Regulus A, making it invisible to amateur telescopes.
Of the brightest stars in the sky, Regulus is closest to the plane of the ecliptic and is regularly obscured by the Moon. Occultation by the planets Mercury and Venus is also possible, but rare, as is occultation by asteroids. The last planetary eclipse (of the planet Venus) of the star Regulus occurred on July 7, 1959. The next one will happen on October 1, 2044 and also by Venus. Other planets will not obscure Regulus for the next few millennia due to their positions.
Adara
The star Adara or Epsilon Canis Majoris is the second brightest star in the constellation Canis Major. With an apparent magnitude of 1.5, Adara is the 22nd brightest star in the sky. Its absolute magnitude is -4.8, and its distance to Earth is approximately 400 light years.
Adara has a spectral class of B2II, a surface temperature of 24,750° Kelvin and a luminosity 20,000 times that of the Sun. The star has a mass of 10 solar masses.
The image above shows an uncropped photograph of the star Adara (North is Up) taken with the Takahashi E-180 Astrograph.
Adara is a double star located 430 light years away from Earth. The main star has a bluish-white color (spectral class B2) with a high surface temperature (25000° K). It emits a total radiation that is 20,000 times greater than that of the Sun. If this star were at the same distance as Sirius, it would outshine all other stars in the sky and would be 15 times brighter than the planet Venus. This star is also one of the most powerful sources of ultraviolet light in the sky. This is a strong source of photons capable of ionizing hydrogen atoms in the interstellar gas near the Sun and is very important in determining the ionization state of the interstellar cloud.
The companion star has an apparent magnitude of 7.5 and is located 7.5 arcseconds from the main star. However, this star can only be seen in large telescopes because the main star is about 250 times brighter than its companion.
Several million years ago, Adara was much closer to the Sun than it is now, causing it to be much brighter in the night sky. About 4,700,000 years ago, Adara was located 34 light-years from the Sun and was a very bright star with an apparent magnitude of -3.99. No other star has reached this brightness since then, and no other star will reach this brightness in the next five million years.
Castor
The star Castor or Alpha Gemini is the second brightest star in the constellation Gemini. With an apparent magnitude of 1.57, Castor is the 23rd brightest star in the sky. Its absolute magnitude is 0.5, and its distance to Earth is 49 light years.
Castor has a spectral class of A1V + A2V, a surface temperature of 10300° Kelvin and a luminosity 30 times greater than that of the Sun. The mass of the star is equal to 2.2 solar masses, and its diameter is 2.3 times greater than that of the Sun.
The image above shows an uncropped photograph of the star Castor (North is Up) taken with the Takahashi E-180 Astrograph.
Visually, the double star Castor was discovered in 1678. Its apparent magnitude is 2.0 and 2.9 (combined magnitude is 1.58). The separated hot white stars (spectral type A) are separated by 6 arcseconds and have an orbital period of 467 years around their common center of mass. Each of the components of this pair is itself a spectroscopic double star, making Castor a quadruple star system. Castor has a faint satellite 72 arcseconds distant from it, but with the same parallax and proper motion. This satellite is a double darkened star system with a period of about 1 day. This binary star system is just one of several in which both components of the pair are M dwarf stars. Castor can thus be considered a six-star system, with six individual stars gravitationally bound to each other.
The twin stars Castor and Pollux are best visible during spring evenings. Unlike real-life twins, Castor and Pollux have little in common. Castor is a white quadruple star composed of fairly similar white components (spectral class A), while Pollux is an orange-colored cool giant (spectral class K0IIIb). Close conjugation with Castor gives Pollux a brighter color.
For many centuries, millions of human eyes, with the onset of night, direct their gaze upward - towards the mysterious lights in the sky - stars of our Universe. Ancient people saw various figures of animals and people in clusters of stars, and each of them created its own story. Later, such clusters began to be called constellations. Today, astronomers identify 88 constellations that divide the starry sky into certain areas by which one can navigate and determine the location of stars. In our Universe, the most numerous objects accessible to the human eye are stars. They represent a source of light and energy for the entire solar system. They also create the heavy elements necessary for the origin of life. And without the stars of the Universe there would be no life, because the Sun gives its energy to almost all living beings on Earth. It warms the surface of our planet, thereby creating a warm oasis full of life among the permafrost of space. The degree of brightness of a star in the Universe is determined by its size.
Do you know the biggest star in the entire Universe?
The star VY Canis Majoris, located in the constellation Canis Major, is the largest representative of the stellar world. At the moment it is the largest star in the Universe. The star is located 5 thousand light years from the solar system. The diameter of the star is 2.9 billion km.
But not all stars in the Universe are so huge. There are also so-called dwarf stars.
Comparative sizes of stars

Astronomers rate the size of stars on a scale according to which the brighter the star, the lower its number. Each subsequent number corresponds to a star ten times less bright than the previous one. The brightest star in the night sky in the Universe is Sirius. Its apparent magnitude is -1.46, meaning it is 15 times brighter than a star with magnitude zero. Stars whose magnitude is 8 or more cannot be seen with the naked eye. Stars are also classified into spectral classes by color, indicating their temperature. There are the following classes of stars in the Universe: O, B, A, F, G, K, and M. Class O corresponds to the hottest stars in the Universe - blue. The coolest stars belong to class M, their color is red.
Class | Temperature,K | true color | Visible color | Main features |
---|---|---|---|---|
O | 30 000—60 000 | blue | blue | Weak lines of neutral hydrogen, helium, ionized helium, multiply ionized Si, C, N. |
B | 10 000—30 000 | white-blue | white-blue and white | Absorption lines of helium and hydrogen. Weak H and K lines of Ca II. |
A | 7500—10 000 | white | white | Strong Balmer series, lines H and K of Ca II intensify towards class F. Also, closer to class F, lines of metals begin to appear |
F | 6000—7500 | yellow-white | white | The H and K lines of Ca II, the lines of metals, are strong. The hydrogen lines begin to weaken. The Ca I line appears. The G band formed by the Fe, Ca and Ti lines appears and intensifies. |
G | 5000—6000 | yellow | yellow | The H and K lines of Ca II are intense. Ca I line and numerous metal lines. The hydrogen lines continue to weaken, and bands of CH and CN molecules appear. |
K | 3500—5000 | orange | yellowish orange | Metal lines and G band are intense. The hydrogen line is almost invisible. TiO absorption bands appear. |
M | 2000—3500 | red | orange-red | The bands of TiO and other molecules are intense. The G band is weakening. Metal lines are still visible. |
Contrary to popular belief, it is worth noting that the stars of the Universe do not actually twinkle. This is just an optical illusion - the result of atmospheric interference. A similar effect can be observed on a hot summer day, looking at hot asphalt or concrete. Hot air rises and it feels like you're looking through shaking glass. The same process causes the illusion of starry twinkling. The closer a star is to Earth, the more it will “twinkle” because its light passes through denser layers of the atmosphere.
Nuclear Hearth of the Universe Stars
A star in the Universe is a giant nuclear center. The nuclear reaction inside it converts hydrogen into helium, thanks to the process of fusion, which is how the star acquires its energy. Hydrogen nuclei with one proton combine to form helium atoms with two protons. The nucleus of an ordinary hydrogen atom has only one proton. Two isotopes of hydrogen also contain one proton, but also have neutrons. Deuterium has one neutron, while tritium has two. Deep inside the star, a deuterium atom combines with a tritium atom to form a helium atom and a free neutron. As a result of this long process, enormous amounts of energy are released.
For main sequence stars, the main source of energy is nuclear reactions involving hydrogen: the proton-proton cycle, characteristic of stars with masses around the Sun, and the CNO cycle, which occurs only in massive stars and only if they contain carbon. At later stages of a star’s life, nuclear reactions can occur with heavier elements, up to iron.
Proton-proton cycle | CNO cycle | |
Basic chains |
|
|
When a star's hydrogen supply is depleted, it begins to convert helium into oxygen and carbon. If the star is massive enough, the conversion process will continue until carbon and oxygen form neon, sodium, magnesium, sulfur and silicon. Eventually, these elements are converted into calcium, iron, nickel, chromium and copper until the core is composed entirely of metal. As soon as this happens, the nuclear reaction will stop because the melting point of iron is too high. The internal gravitational pressure becomes higher than the external pressure of the nuclear reaction and, eventually, the star collapses. The further development of events depends on the initial mass of the star.
Types of stars in the Universe
The main sequence is the period of existence of stars in the Universe, during which a nuclear reaction takes place inside it, which is the longest period of a star’s life. Our Sun is currently in this period. During this time, the star undergoes slight fluctuations in brightness and temperature. The duration of this period depends on the mass of the star. In large massive stars it is shorter, and in small ones it is longer. Very large stars have internal fuel that lasts for several hundred thousand years, while small stars like the Sun will shine for billions of years. The largest stars turn into blue giants during the main sequence.
Types of stars in the Universe |
|
Red giant is a large star of reddish or orange color. It represents the late stage of the cycle when hydrogen supplies are running low and helium begins to be converted into other elements. An increase in the internal temperature of the core leads to the collapse of the star. The outer surface of the star expands and cools, causing the star to turn red. Red giants are very large. Their size is a hundred times larger than ordinary stars. The largest of the giants turn into red supergiants. A star called Betelgeuse in the constellation Orion is the brightest example of a red supergiant. | ![]() |
White dwarf- this is what remains of an ordinary star after it goes through the red giant stage. When a star has no more fuel left, it can release some of its matter into space, forming a planetary nebula. What remains is a dead core. A nuclear reaction is not possible in it. It shines due to its remaining energy, but sooner or later it runs out, and then the core cools down, turning into a black dwarf. White dwarfs are very dense. They are no larger in size than the Earth, but their mass can be compared to the mass of the Sun. These are incredibly hot stars, with temperatures reaching 100,000 degrees or more. | ![]() |
Brown dwarf also called a substar. During their life cycle, some protostars never reach a critical mass to begin nuclear processes. If the mass of a protostar is only 1/10 the mass of the Sun, its radiance will be short-lived, after which it will quickly fade. What remains is a brown dwarf. It's a massive ball of gas, too big to be a planet and too small to be a star. It is smaller than the Sun, but several times larger than Jupiter. Brown dwarfs emit neither light nor heat. This is just a dark clot of matter existing in the vastness of the Universe. | ![]() |
Cepheid is a star with variable luminosity, the pulsation cycle of which ranges from a few seconds to several years, depending on the type of variable star. Cepheids typically change their luminosity at the beginning of their lives and at the end of their lives. They are internal (changing luminosity due to processes inside the star) and external, changing brightness due to external factors, such as the influence of the orbit of a nearby star. This is also called a dual system. | ![]() |
Many stars in the Universe are part of large star systems. Double stars is a system of two stars gravitationally bound to each other. They rotate in closed orbits around one center of mass. It has been proven that half of all the stars in our galaxy have a pair. Visually, paired stars look like two separate stars. They can be determined by the shift of spectrum lines (Doppler effect). In eclipsing binary systems, stars periodically eclipse each other because their orbits are located at a small angle to the line of sight. | ![]() |
Life Cycle of Stars in the Universe
A star in the Universe begins its life as a cloud of dust and gas called a nebula. The gravity of a nearby star or the blast wave from a supernova can cause the nebula to shrink. Elements of the gas cloud coalesce into a dense region called a protostar. As a result of subsequent compression, the protostar heats up. Eventually, it reaches critical mass and the nuclear process begins; Gradually the star goes through all the phases of its existence. The first (nuclear) stage of a star's life is the longest and most stable. The lifespan of a star depends on its size. Large stars use up their vital fuel faster. Their life cycle can last no more than several hundred thousand years. But small stars live for many billions of years, as they spend their energy more slowly.
But, be that as it may, sooner or later, the stellar fuel runs out, and then the small star turns into a red giant, and the large star into a red supergiant. This phase will last until the fuel is completely used up. At this critical moment, the internal pressure of the nuclear reaction will weaken and can no longer balance the force of gravity, and as a result, the star will collapse. Small stars in the universe then typically develop into a planetary nebula with a bright, shining core called a white dwarf. Over time, it cools down, turning into a dark clot of matter - a black dwarf.
For big stars, things happen a little differently. During the collapse, they release incredible amounts of energy, and a powerful explosion gives birth to a supernova. If its magnitude is 1.4 solar magnitudes, then, unfortunately, the core will not be able to maintain its existence and, after the next collapse, the supernova will become neutron. The internal matter of the star will compress to such an extent that the atoms form a dense shell consisting of neutrons. If the stellar magnitude is three times the solar magnitude, then the collapse will simply destroy it, erase it from the face of the Universe. All that will remain of it is an area of strong gravity, nicknamed a black hole.
The nebula left behind by a star in the Universe can expand over millions of years. In the end, it will be affected by the gravity of a neighboring star or the blast wave of a supernova and everything will happen again. This process will occur throughout the Universe - an endless cycle of life, death and rebirth. The result of this stellar evolution is the formation of heavy elements necessary for life. Our solar system originated from the second or third generation of the nebula, and due to this, there are heavy elements on Earth and other planets. This means that there are pieces of stars in each of us. All the atoms of our body were born in an atomic source or as a result of a destructive supernova explosion
.
Stars can be very different: small and large, bright and not very bright, old and young, hot and “cold”, white, blue, yellow, red, etc.
The Hertzsprung–Russell diagram allows you to understand the classification of stars.
It shows the relationship between the absolute magnitude, luminosity, spectral type and surface temperature of the star. The stars in this diagram are not located randomly, but form clearly visible areas.
Most of the stars are on the so-called main sequence. The existence of the main sequence is due to the fact that the hydrogen burning stage makes up ~90% of the evolutionary time of most stars: the burning of hydrogen in the central regions of the star leads to the formation of an isothermal helium core, the transition to the red giant stage and the departure of the star from the main sequence. The relatively short evolution of red giants leads, depending on their mass, to the formation of white dwarfs, neutron stars or black holes.
Being at various stages of their evolutionary development, stars are divided into normal stars, dwarf stars, and giant stars.
Normal stars are main sequence stars. These include our Sun. Sometimes normal stars like the Sun are called yellow dwarfs.
Yellow dwarf
A yellow dwarf is a type of small main sequence star with a mass between 0.8 and 1.2 solar masses and a surface temperature of 5000–6000 K.
The lifespan of a yellow dwarf is on average 10 billion years.
After the entire supply of hydrogen burns, the star increases in size many times and turns into a red giant. An example of this type of star is Aldebaran.
The red giant ejects its outer layers of gas to form planetary nebulae, while the core collapses into a small, dense white dwarf.
A red giant is a large star with a reddish or orange color. The formation of such stars is possible both at the stage of star formation and at later stages of their existence.
At an early stage, the star radiates due to the gravitational energy released during compression, until the compression is stopped by the thermonuclear reaction that has begun.
In the later stages of the evolution of stars, after the burning of hydrogen in their cores, the stars leave the main sequence and move to the region of red giants and supergiants of the Hertzsprung-Russell diagram: this stage lasts approximately 10% of the time of the “active” life of stars, that is, the stages of their evolution , during which nucleosynthesis reactions occur in the stellar interior.
The giant star has a relatively low surface temperature, about 5000 degrees. A huge radius, reaching 800 solar and due to such large sizes, enormous luminosity. The maximum radiation occurs in the red and infrared regions of the spectrum, which is why they are called red giants.
The largest of the giants turn into red supergiants. A star called Betelgeuse in the constellation Orion is the most striking example of a red supergiant.
Dwarf stars are the opposite of giants and may be next.
A white dwarf is what remains of an ordinary star with a mass of less than 1.4 solar masses after it passes through the red giant stage.
Due to the absence of hydrogen, thermonuclear reactions do not occur in the core of such stars.
White dwarfs are very dense. They are no larger in size than the Earth, but their mass can be compared to the mass of the Sun.
These are incredibly hot stars, their temperatures reach 100,000 degrees or more. They shine using their remaining energy, but over time it runs out and the core cools, turning into a black dwarf.
Red dwarfs are the most common stellar-type objects in the Universe. Estimates of their numbers range from 70 to 90% of all stars in the galaxy. They are quite different from other stars.
The mass of red dwarfs does not exceed a third of the solar mass (the lower limit of mass is 0.08 solar, followed by brown dwarfs), the surface temperature reaches 3500 K. Red dwarfs have a spectral class of M or late K. Stars of this type emit very little light, sometimes 10,000 times smaller than the Sun.
Given their low radiation, none of the red dwarfs are visible from Earth with the naked eye. Even the closest red dwarf to the Sun, Proxima Centauri (the closest star in the triple system to the Sun), and the nearest single red dwarf, Barnard's Star, have apparent magnitudes of 11.09 and 9.53, respectively. In this case, a star with a magnitude of up to 7.72 can be observed with the naked eye.
Due to the low rate of hydrogen combustion, red dwarfs have very long lifespans, ranging from tens of billions to tens of trillions of years (a red dwarf with a mass of 0.1 solar masses will burn for 10 trillion years).
In red dwarfs, thermonuclear reactions involving helium are impossible, so they cannot turn into red giants. Over time, they gradually shrink and heat up more and more until they use up the entire supply of hydrogen fuel.
Gradually, according to theoretical concepts, they turn into blue dwarfs - a hypothetical class of stars, while none of the red dwarfs have yet managed to turn into a blue dwarf, and then into white dwarfs with a helium core.
Brown dwarf - substellar objects (with masses ranging from about 0.01 to 0.08 solar masses, or, respectively, from 12.57 to 80.35 Jupiter masses and a diameter approximately equal to the diameter of Jupiter), in the depths of which, in contrast from main sequence stars, there is no thermonuclear fusion reaction with the conversion of hydrogen into helium.
The minimum temperature of main sequence stars is about 4000 K, the temperature of brown dwarfs lies in the range from 300 to 3000 K. Brown dwarfs constantly cool down throughout their lives, and the larger the dwarf, the slower it cools.
Subbrown dwarfs
Subbrown dwarfs or brown subdwarfs are cool formations that fall below the brown dwarf limit in mass. Their mass is less than approximately one hundredth the mass of the Sun or, accordingly, 12.57 the mass of Jupiter, the lower limit is not determined. They are generally considered to be planets, although the scientific community has not yet come to a final conclusion about what is considered a planet and what is a sub-brown dwarf.
Black dwarf
Black dwarfs are white dwarfs that have cooled and, as a result, do not emit in the visible range. Represents the final stage of the evolution of white dwarfs. The masses of black dwarfs, like the masses of white dwarfs, are limited above 1.4 solar masses.
A binary star is two gravitationally bound stars orbiting a common center of mass.
Sometimes there are systems of three or more stars, in this general case the system is called a multiple star.
In cases where such a star system is not too far from the Earth, individual stars can be distinguished through a telescope. If the distance is significant, then it is possible to understand that astronomers can see a double star only by indirect signs - fluctuations in brightness caused by periodic eclipses of one star by another and some others.
New star
Stars whose luminosity suddenly increases 10,000 times. The nova is a binary system consisting of a white dwarf and a companion star located on the main sequence. In such systems, gas from the star gradually flows to the white dwarf and periodically explodes there, causing a burst of luminosity.
Supernova
A supernova is a star that ends its evolution in a catastrophic explosive process. The flare in this case can be several orders of magnitude larger than in the case of a nova. Such a powerful explosion is a consequence of the processes occurring in the star at the last stage of evolution.
Neutron star
Neutron stars (NS) are stellar formations with masses of the order of 1.5 solar and sizes noticeably smaller than white dwarfs; the typical radius of a neutron star is presumably on the order of 10-20 kilometers.
They consist mainly of neutral subatomic particles - neutrons, tightly compressed by gravitational forces. The density of such stars is extremely high, it is comparable, and according to some estimates, can be several times higher than the average density of the atomic nucleus. One cubic centimeter of NS substance will weigh hundreds of millions of tons. The gravity on the surface of a neutron star is about 100 billion times higher than on Earth.
In our Galaxy, according to scientists, there may exist from 100 million to 1 billion neutron stars, that is, somewhere around one per thousand ordinary stars.
Pulsars
Pulsars are cosmic sources of electromagnetic radiation coming to Earth in the form of periodic bursts (pulses).
According to the dominant astrophysical model, pulsars are rotating neutron stars with a magnetic field that is inclined to the rotation axis. When the Earth falls into the cone formed by this radiation, it is possible to detect a pulse of radiation repeating at intervals equal to the revolution period of the star. Some neutron stars rotate up to 600 times per second.
Cepheids
Cepheids are a class of pulsating variable stars with a fairly precise period-luminosity relationship, named after the star Delta Cephei. One of the most famous Cepheids is Polaris.
The given list of the main types (types) of stars with their brief characteristics, of course, does not exhaust the entire possible variety of stars in the Universe.