Experiments of Ioffe and Millikan. Electron. Presentation on the topic "Physics A. F. Ioffe and R. E. Millikan. Their life path. The Ioffe - Millikan experience"
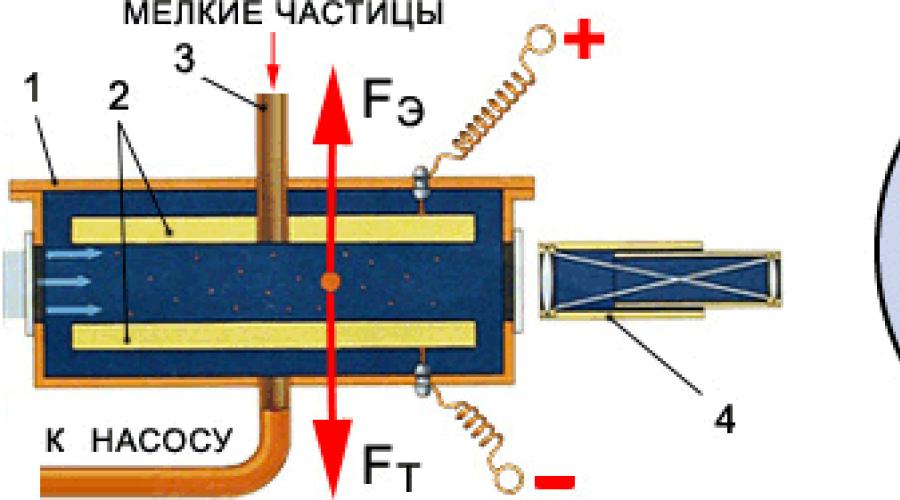
Read also
Prepared by Class 11-A student, Kosh No. 125 Kristina Konovalova
Slide 2
The Ioffe-Milliken experiment Abram Fedorovich Ioffe Robert AndrewsMilliken
Slide 3
Ioffe-Millikan experiment
By the end of the 19th century, in a number of very diverse experiments, it was established that there is a certain negative charge carrier, which was called an electron. However, this was actually a hypothetical unit, since, despite the abundance practical material, not a single experiment involving a single electron has been carried out. It was not known whether there are varieties of electrons for different substances or whether they are always the same, what charge an electron carries, or whether a charge can exist separately from a particle. In general, there were heated debates about the electron in the scientific community, but there was no sufficient practical basis that would definitely stop all the debate.
Slide 4
The figure shows a diagram of the installation used in the experiment of A.F. Ioffe. In a closed vessel, the air from which was pumped out to a high vacuum, there were two metal plates P, located horizontally. From chamber A, through hole O, small charged zinc dust particles entered the space between the plates. These dust particles were observed under a microscope.
Slide 5
So, charged dust particles and droplets in a vacuum will fall from the top plate to the bottom, but this process can be stopped if the top plate is charged positively and the bottom plate negatively. The emerging electric field will act with Coulomb forces on charged particles, preventing them from falling. By adjusting the amount of charge, they ensured that the dust particles floated in the middle between the plates. Next, the charge of dust particles or droplets was reduced by irradiating them with X-rays or ultraviolet light. Losing their charge, the dust particles began to fall again, they were stopped again by adjusting the charge of the plates. This process was repeated several times, calculating the charge of droplets and dust particles using special formulas. As a result of these studies, it was possible to establish that the charge of dust particles or drops always changed abruptly, by a strictly defined value, or by a size that was a multiple of this value.
Slide 6
Abram Fedorovich Ioffe
Abram Fedorovich Ioffe is a Russian physicist who made many fundamental discoveries and conducted a huge amount of research, including in the field of electronics. He conducted research on the properties semiconductor materials, discovered the rectifying property of the metal-insulator transition, which was subsequently explained using the theory of the tunnel effect, suggested the possibility of converting light into electricity.
Slide 7
Abram Fedorovich was born on October 14, 1980 in the city of Romny, Poltava province (now Poltava region, Ukraine) in the family of a merchant. Since Abram's father was a fairly rich man, he did not stint giving a good education to his son. In 1897, Ioffe received secondary education at a real school. hometown. In 1902, he graduated from the St. Petersburg Institute of Technology and entered the University of Munich in Germany. In Munich he works under the guidance of Wilhelm Conrad Roentgen himself. Wilhelm Conrad, seeing the student’s diligence and not any talent, tries to persuade Abram to stay in Munich and continue scientific activity, but Ioffe turned out to be a patriot of his country. After graduating from university in 1906, receiving academic degree Ph.D., he returns to Russia.
Slide 8
In Russia, Ioffe gets a job in Polytechnical Institute. In 1911, he experimentally determined the value of the electron charge using the same method as Robert Millikan (metal particles were balanced in electric and gravitational fields). Due to the fact that Ioffe published his work only two years later, the glory of discovering the measurement of the electron charge went to the American physicist. In addition to determining the charge, Ioffe proved the reality of the existence of electrons independently of matter, investigated the magnetic effect of the flow of electrons, and proved the static nature of the emission of electrons during the external photoelectric effect.
Slide 9
In 1913, Abram Fedorovich defended his master's thesis, and two years later his doctorate thesis in physics, which was a study of the elastic and electrical properties of quartz. In the period from 1916 to 1923, he actively studied the mechanism of electrical conductivity of various crystals. In 1923, it was on Ioffe’s initiative that basic research and studying the properties of materials that were completely new at that time - semiconductors. The first work in this area was carried out with the direct participation of a Russian physicist and concerned the analysis of electrical phenomena between a semiconductor and a metal. He discovered the rectifying property of the metal-semiconductor transition, which was only substantiated 40 years later using the theory of the tunnel effect.
Slide 10
While studying the photoelectric effect in semiconductors, Ioffe expressed a rather bold idea at that time that in a similar way it would be possible to convert light energy into electric current. This became a prerequisite for the subsequent creation of photoelectric generators, and in particular silicon converters, subsequently used in solar panels. Together with his students, Abram Fedorovich creates a classification system for semiconductors, as well as a method for determining their basic electrical and physical properties. In particular, the study of their thermoelectric properties subsequently became the basis for the creation of semiconductor thermoelectric refrigerators, widely used throughout the world in the fields of radio electronics, instrument making and space biology.
Slide 11
Abram Fedorovich Ioffe made a huge contribution to the formation and development of physics and electronics. He was a member of many Academies of Sciences (Berlin and Göttingen, American, Italian), as well as honorary members of many universities around the world. He was awarded many awards for his achievements and research. Abram Fedorovich died on October 14, 1960.
Slide 12
Milliken Robert Andrews
American physicist Robert Millikan was born in Morrison (Illinois) on March 22, 1868 in the family of a priest. After graduation high school Robert enters Oberlin College in Ohio. There his interests focused on mathematics and ancient Greek. To earn money, he taught physics in college for two years. 1891 Millikan received a bachelor's degree, and 1893 a master's degree in physics.
Slide 13
At Columbia University, Millikan studied under the guidance of famous physicist M.I.Pupin. He spent one summer at the University of Chicago, where he worked under the guidance of the famous experimental physicist Albert Abraham Michelson.
Slide 14
In 1895, he defended his doctoral dissertation at Columbia University on the study of the polarization of light. Millikan spent the next year in Europe, where he met with Henri Becquerel, Max Planck, Walter Nernst, and A. Poincaré.
Slide 15
1896 Millikan returned to the University of Chicago, where he became Michelson's assistant. Over the next twelve years, Millikan wrote several physics textbooks that were adopted as textbooks for colleges and high schools (with additions, they remained so for over 50 years). 1910 Millikan was appointed professor of physics.
Slide 16
Robert Millikan developed the droplet method, which made it possible to measure the charge of individual electrons and protons (1910 - 1914) a large number of experiments to accurately calculate the charge of an electron. Thus, he experimentally proved the discreteness of the electric charge and for the first time quite accurately determined its value (4.774 * 10^-10 electrostatic units). I checked Einstein's equation for the photoelectric effect in the visible and ultraviolet rays, determined Planck's constant (1914).
Slide 17
1921 Millikan was appointed director of the new Bridges Physical Laboratory and head of the executive committee of the California Institute of Technology. Here he carried out a large series of studies of cosmic rays, in particular experiments (1921 - 1922) with air sheaves with recording electroscopes at altitudes of 15500 m. 1923 Millikan was awarded the Nobel Prize in Physics “for his work on the determination of the elementary electric charge and the photoelectric effect "
Slide 18
During 1925-1927 Millikan demonstrated that the ionizing effect of cosmic radiation decreases with depth and confirmed extraterrestrial origin these "cosmic rays". Studying the trajectories of cosmic particles, he identified alpha particles, fast electrons, protons, neutrons, positrons and gamma quanta in them. Independently of Vernov, he discovered the latitude effect of cosmic rays in the stratosphere.
View all slides
The phenomenon of interaction of a charged body with an electric field was used by the American physicist Robert Mil-liken to confirm the discreteness of the electric charge and measure its smallest value. Over the course of 1906–1916, he conducted a series of experiments that were noted for their originality and great accuracy. In accordance with the purpose and strategy of the study, it was necessary to find a way to measure forces of the order of 10 -13 N acting on particles weighing 10 -15 kg.
Diagram of the research facility R. Milliken shown in Fig. 4.17.
Robert Andrews Milliken (1868 — 1953) — American physicist, investigated the properties of the electron, was the first to measure the charge of the electron, studied the phenomena of the photoelectric effect, ultraviolet radiation, cosmic radiation, atomic structure.
In hermetically sealed closed chamber, protecting the installation from external influences, placed round brass plates A and B with a diameter of 22 cm. The distance between them was 1.6 cm. The installation included system C, injecting into the space between the plates mineral oil, which formed a cloud of droplets with a diameter of 10 -4 cm. A special system in right moment created an electric field between the plates with a intensity of about 10 6 N/C.
Drops of oil falling into the space between the plates were illuminated by a strong light source. Perpendicular to the direction of the rays through microscope D, it was possible to observe the movement of oil droplets. A scale placed in the field of view of the microscope made it possible to count the path traveled by the drop over a certain time interval.
Mineral oil was chosen because it evaporates very slowly and the mass of the droplet remains practically unchanged for a long time.
The idea of the study by R. Milliken Briefly it can be formulated as follows:
measure a large number of changes in the electric charge of a droplet and find a certain pattern in these changes.
To solve the problem, the movement of a droplet isolated in the field of view of the microscope was considered.
Immediately after spraying, the droplet begins to accelerate downwards under the influence of gravity. At the same time, it acquires a certain charge, and the resistance force, proportional to the speed, gradually increases. When equilibrium is established between the force of gravity and the force of air resistance (Fig. 4.18), the droplet begins to move uniformly, in accordance with the equation mg—kv 1 = 0.
Here k— proportionality coefficient, which takes into account the influence of air on the movement of the droplet. Material from the site
After applying voltage to the plates, an electric force appears, the action of which leads to a change in the speed of movement of the droplet. Changing tension electric field between the plates, it was possible to ensure that the droplet began to move upward evenly (Fig. 4.19). The steady-state speed was determined from the equation of motion, which also takes into account the electric force
mg—qE +kv 2 = 0.
The joint solution of the two equations gave the value of the droplet charge:
q = k(v 1 + v 2) / E.
In the course of further experiments, the droplet was illuminated with an ultraviolet or X-ray beam. At the same time, its charge changed abruptly. Analysis of the measured charge values allowed us to establish with certain certainty that the change in the charge of the droplet was not less than 1.6. 10 -19 Grade. An electron has such a charge.
Results obtained Milliken, were confirmed in experimental studies by scientists in France, Germany, England, and Russia.
On this page there is material on the following topics:
GD study of the phenomenon of photoelectric effect
Millikan installation
Robert Millikan brief physical law
Millikan formulas
What is Robert Millikan's law of physics?
Questions about this material:
By the beginning of the 20th century. the existence of electrons was established in a number of independent experiments. But, despite the enormous experimental material accumulated by various scientific schools, the electron remained, strictly speaking, a hypothetical particle. The reason is that there has not been a single experiment involving single electrons.
Electrons first appeared as a convenient hypothesis to explain the laws of electrolysis, then they were discovered in a gas discharge, which confirmed their existence in all bodies. However, it was not clear whether physics was dealing with the same electron, the same for all substances and bodies, or whether the properties of the electron represented the average characteristics of a wide variety of “brother electrons.”
To answer this question, in 1910-1911, the American scientist Robert Andrews Millikan and the Soviet physicist Abram Fedorovich Ioffe independently carried out precise experiments in which it was possible to observe single electrons.
In their experiments, in a closed vessel 1, the air from which was evacuated by a pump to a high vacuum, there were two horizontally located metal plates 2. Between them, through a tube 3, a cloud of charged metal dust particles or oil droplets was placed. They were observed through a microscope 4 with a special scale, which made it possible to observe their settling (falling) down.
Let us assume that the dust particles or droplets were negatively charged before being placed between the plates. Therefore, their subsidence (fall) can be stopped if the bottom plate is charged negatively and the top plate positively. This is what they did, achieving the equilibrium of the dust particle (droplet), which was observed through a microscope.
Then the charge of the dust particles (droplets) was reduced by exposing them to ultraviolet or X-ray radiation. The dust particles (droplets) began to fall as the supporting electrical force decreased. By imparting an additional charge to the metal plates and thereby increasing the electric field, the dust particle was stopped again. This was done several times, each time using a special formula to calculate the charge of dust particles.
The experiments of Millikan and Ioffe showed that the charges of drops and dust particles always change abruptly. The minimum “portion” of electric charge is elementary electric charge, equal to e = 1.6·10-19 C. However, the charge of the dust particle does not leave on its own, but along with the particle of matter. Consequently, in nature there is a particle of matter that has the smallest charge, then an indivisible charge - the charge of an electron. Thanks to the Ioffe-Millikan experiments, the existence of the electron turned from a hypothesis into a scientifically proven fact.
There is currently evidence of the existence elementary particles(quarks) with fractional electric charges equal to 1/Ze and 2/Ze. However, the electric charge of any body is always an integer multiple of the elementary electric charge; Other “portions” of electric charge capable of moving from one body to another have not yet been experimentally discovered in nature.
Scientists are well aware that this particle is the fundamental component of everything material world. Accordingly, the question arose about studying and measuring its properties. The first precision measurement of the electric charge of an electron was achieved by Robert Millikan. His experimental setup was a large and capacious flat capacitor consisting of two metal plates with a camera between them. Millikan applied a constant voltage to the capacitor plates from powerful battery, creating a high potential difference on them, and between the plates he placed finely sprayed drops - first of water, and then of oil, which, as it turned out, behaves in an electrostatic field much more stable, and most importantly - evaporates much more slowly. First, Millikan measured the limiting speed of falling drops - that is, the speed at which the force of gravity acting on the drops is balanced by the force of air resistance. From this speed, the scientist determined the volume and mass of droplets of aerosol suspension. After that, he sprayed an identical aerosol in the presence of an electrostatic field, that is, with a battery connected. In this case, the oil droplets remained suspended for a long time, since the forces of gravitational attraction of the Earth were balanced by the forces of electrostatic repulsion between the aerosol droplets.
The reason why oil aerosol droplets become electrified is simple: it is a simple electrostatic charge, similar to that, which accumulates, say, on clothes that we take out of the spin dryer as a result of the fact that fabric rubs against fabric - it arises as a result of the friction of droplets with the air filling the chamber. However, due to the microscopic size of the oil droplets in the chamber, they cannot receive a large charge, and the amount of charge on the droplets will be a multiple of the unit charge of the electron. This means that by gradually lowering the external voltage, we will observe how drops of oil periodically “precipitate”, and by the gradations of the voltage scale at which the next portion of the aerosol is deposited, we can judge absolute value unit charge, since electrified drops cannot carry a fractional charge.
In addition, Millikan irradiated the oil suspension with X-rays and additionally ionized its organic molecules in order to increase their electrification and extend the time of experimental observation, while simultaneously increasing the voltage in the chamber, and did this many times to refine the data obtained. Finally, having accumulated enough experimental data for statistical processing, Millikan calculated the value of the unit charge and published the results, which contained the calculated electron charge as accurately as possible for those years.
Millikan's experiment was extremely labor-intensive. The scientist had, in particular, to constantly measure and take into account air humidity and Atmosphere pressure- and so on throughout the five years of continuous monitoring of your installation. The reward for titanic work was Nobel Prize in Physics for 1923, awarded to Millikan for his 1913 publication. It is interesting that for all the apparent simplicity of Millikan’s camera, it did not become a museum exhibit. Already in the 1960s, when the quark hypothesis appeared ( cm. Standard Model), modern, improved installations were built, operating on the principle described above, on which scientists unsuccessfully searched free quarks. Since it was not possible to detect any (quarks various types must have electric charges equal to 1/3 and 2/3 of the electron charge), this served as additional confirmation of the theory according to which quarks in free form in modern nature do not meet and are always in a bound state inside other elementary particles.
Robert Andrews Millikan, 1868-1953
American physicist. Born in Morrison, Illinois, into the family of a Congregational minister and a parochial girls' school teacher. After graduating from Oberlin College in Ohio, he taught for a while Greek language and, part-time, physics in primary school. Fascinated by the latter, he entered the Faculty of Physics at Columbia University, after which he completed a year-long internship in leading laboratories in Europe, and then joined the teaching staff of the University of Chicago. There he received universal recognition as an authoritative teacher (in particular, long years Physics was taught in American schools using his textbooks). There, in Chicago, he conducted his famous experiment for a number of years, which made it possible for the first time to determine the charge of an electron with sufficient accuracy and put Millikan in the forefront of representatives of American science. At the same time, the scientist was engaged in active social activities and, to some extent, contributed to the formation of a new image of a socially active intellectual in the minds of the mass reader.
During World War I, with the rank of colonel, Milliken led the US Signal Corps. The scientist devoted a lot of time to organizing research institutions and in 1921 actually headed the newly created California Institute of Technology in Pasadena. At the same time, Millikan did not leave and research activities, being one of the pioneers of cosmic ray physics. As a result, he became the personified symbol of his generation of scientists, continuing the traditions of the Englishmen John Tyndall and Michael Faraday, and anticipated the emergence of such outstanding scientific popularizers as Carl Sagan.
The idea of discreteness of electric charge was first expressed by B. Franklin in 1752. Experimentally, the discreteness of charges was justified by the laws of electrolysis, discovered by M. Faraday in 1834. Numerical value elementary charge (the smallest electrical charge found in nature) was theoretically calculated based on the laws of electrolysis using Avogadro's number. Direct experimental measurement of the elementary charge was carried out by R. Millikan in classical experiments performed in 1908 - 1916. These experiments also provided irrefutable evidence atomism of electricity.
According to the basic concepts of electronic theory, the charge of a body arises as a result of a change in the number of electrons contained in it (or positive ions, the charge value of which is a multiple of the charge of the electron). Therefore, the charge of any body must change abruptly and in such portions that contain an integer number of electron charges.
All physicists were interested in the magnitude of the electric charge of an electron, and, nevertheless, it has not yet been possible to measure it. Many attempts to make this crucial measurement had already been made by J. J. Thomson, but after ten years of work, Thomson's assistant G. Wilson reported that after eleven various measurements they got eleven different results.
Before starting research with his own method, Millikan conducted experiments using the method used at the University of Cambridge. The theoretical part of the experiment was as follows: body weight was determined by measuring the pressure produced by the body under the influence of gravity on a scale. If an infinitesimal particle of matter is given an electric charge, and if an upward electrical force is applied equal to the downward force of gravity, the particle will be in a state of equilibrium, and the physicist can calculate the magnitude of the electric charge. If in in this case the particle will be given the electric charge of one electron, and it will be possible to calculate the magnitude of this charge.
The Cambridge theory was quite logical, but physicists could not create a device with which they could study individual particles of substances. They had to be content with observing the behavior of a cloud of water droplets charged with electricity. In the chamber, from which the air was partially removed, a vapor cloud was created. Current was supplied to the top of the chamber. After a certain time, the droplets of fog in the cloud calmed down. Then X-rays were passed through the fog, and the water drops received an electric charge.
At the same time, the researchers believed that the electric force directed upward to the one located under high voltage The lid of the chamber is supposed to keep drops from falling. However, in reality none of the difficult conditions, at which, and only at which, the particles could be in a state of equilibrium.
Millikan started looking new way solving the problem.
The method is based on the study of the movement of charged oil droplets in a uniform electric field of known strength E.
Figure 15.2 Experimental setup diagram: P – drop sprayer; K – capacitor; IP – power source; M – microscope; hn – radiation source; P – table surface.
A diagram of one of Millikan’s installations is shown in Fig. 15.1. Millikan measured the electric charge concentrated on individual small spherical droplets that were formed by a sprayer P and acquired an electrical charge by electrification by friction against the walls of the sprayer. Through a small hole in the upper plate of the flat capacitor K, they entered the space between the plates. The movement of the drop was observed through a microscope M.
In order to protect droplets from convection air currents, the condenser is enclosed in protective cover, the temperature and pressure in which are maintained constant. When performing experiments, it is necessary to observe the following requirements:
A. drops must be microscopic in size so that the forces acting on the drop in different directions(up and down) were comparable in magnitude;
b. the charge of the drop, as well as its changes during irradiation (using an ionizer) were equal to a fairly small number of elementary charges. This makes it easier to establish the multiple of the drop's charge to the elementary charge;
V. the droplet density r must be greater than the density of the viscous medium r0 in which it moves (air);
d. The mass of the drop should not change during the entire experiment. To do this, the oil that makes up the drop should not evaporate (oil evaporates much slower than water).
If the capacitor plates were not charged (electric field strength E = 0), then the drop fell slowly, moving from the top plate to the bottom. As soon as the capacitor plates were charged, changes occurred in the movement of the drop: in the case of a negative charge on the drop and a positive charge on the top plate of the capacitor, the fall of the drop slowed down, and at some point in time it changed the direction of movement to the opposite - it began to rise to the top plate.
Determination of elementary charge through a computational experiment.
Knowing the speed of falling of a drop in the absence of an electrostatic field (its charge did not play a role) and the speed of falling of a drop in a given and known electrostatic field, Millikan could calculate the charge of the drop.
Due to viscous resistance, the drop almost immediately after the start of movement (or a change in movement conditions) acquires a constant (steady) speed and moves uniformly. Due to this A= 0, and the speed of the drop can be found. Let us denote the modulus of steady velocity in the absence of an electrostatic field – v g, then:
v g = (m – m 0) g/k (16.5).
If you close electrical circuit capacitor (Figure 1), it will charge and an electrostatic field will be created in it E. In this case, the charge will be subject to an additional force q· E, directed upwards. Newton's law in projection onto the X axis and taking into account that a = 0, will take the form:
-(m – m0) g + q E – k vE = 0 (16.6)
vE = (q·E – (m – m0)·g/k (16.7),
where vE is the steady-state speed of the oil drop in the electrostatic field of the capacitor; v E > 0, if the drop moves upward, v E< 0, если капля движется вниз. Отсюда следует что
q = (vE + |vg|) k/E (16.8),
it follows that by measuring steady-state velocities in the absence of an electrostatic field vg and in its presence vE, it is possible to determine the charge of a drop if the coefficient k = 6·p·h·r is known.
It would seem that to find k it is enough to measure the radius of the drop (the viscosity of air is known from other experiments). However, its direct measurement using a microscope is impossible. The radius of the drop is of the order of magnitude r = 10 -4 – 10 -6 cm, which is comparable in order of magnitude to the light wavelength. Therefore, the microscope provides only a diffraction image of the drop, not allowing its actual dimensions to be measured.
Information about the radius of a drop can be obtained from experimental data on its motion in the absence of an electrostatic field. Knowing v g and taking into account that
m – m 0 = (r – r 0) 4 p r 3 /3 (16.9),
where r is the density of the oil drop,
r = ((9 h v g)/) 1/2 . (16.10).
In his experiments, Millikan changed the charge of a drop by bringing a piece of radium to a capacitor. In this case, the radium radiation ionized the air in the chamber (Fig. 1), as a result of which the drop could capture an additional positive or negative charge. If before this the drop was negatively charged, then it is clear that it is more likely to attach positive ions to itself. On the other hand, due to thermal movement It is possible that negative ions may also join as a result of collisions with them. In both cases, the charge of the drop will change and - stepwise - the speed of its movement v E ". The value q" of the changed charge of the drop in accordance with (16.10) is given by the relation:
q" = (|v g | + v E ") k/E (16.11).
From (1) and (3) the magnitude of the charge attached to the drop is determined:
Dq = |q – q"| = k·|v E – v E "|/E = k·(|Dv E |/E) (16.12).
By comparing the charge values of the same drop, it was possible to verify that the change in charge and the charge of the drop itself are multiples of the same value e 0 - the elementary charge. In his numerous experiments, Millikan received different meanings charges q and q", but they always represented a multiple of the value e 0 = 1.7 . 10 -19 C, that is, q = n·e 0, where n is an integer. From this Millikan concluded that the value e 0 represents the smallest amount of electricity possible in nature, that is, a “portion” or an atom of electricity. Observation of the movement of the same drop, i.e. Millikan repeated its movement downwards (in the absence of an electric field) and up (in the presence of an electric field) in each experiment many times, turning the electric field on and off in a timely manner. The accuracy of measuring the charge of a drop significantly depends on the accuracy of measuring the speed of its movement.
Having experimentally established the discrete nature of the change in electric charge, R. Millikan was able to obtain confirmation of the existence of electrons and determine the value of the charge of one electron (elementary charge) using the oil drop method.
Modern meaning"atom" of electricity e 0 = 1.602 . 10 -19 Grade. This quantity is the elementary electric charge, the carriers of which are electrons e 0 = – 1.602 . 10 -19 C and proton e 0 = +1.602 . 10 -19 Grade. Millikan's work made a huge contribution to physics and gave a huge impetus to the development of scientific thought in the future.
1. What is the essence of Thomson's method?
2. Experimental setup?
3. Thomson tube?
4. Derivation of the formula for the ratio of charge to particle mass?
5. What is the main task of electron and ion optics? And what are they usually called?
6. When was the “magnetic focusing method” discovered?
7. What is its essence?
8. How is the specific charge of an electron determined?
9. Draw an installation diagram based on Millikan’s experience?
10. What requirements must be observed when performing the experiment?
11. Determination of elementary charge through a computational experiment?
12. Derivation of the formula for the charge of a drop through the speed of falling of the drop?
13. Modern meaning of the "atom" of electricity?