Mechanical wave transfers. Waves. General properties of waves. Wave
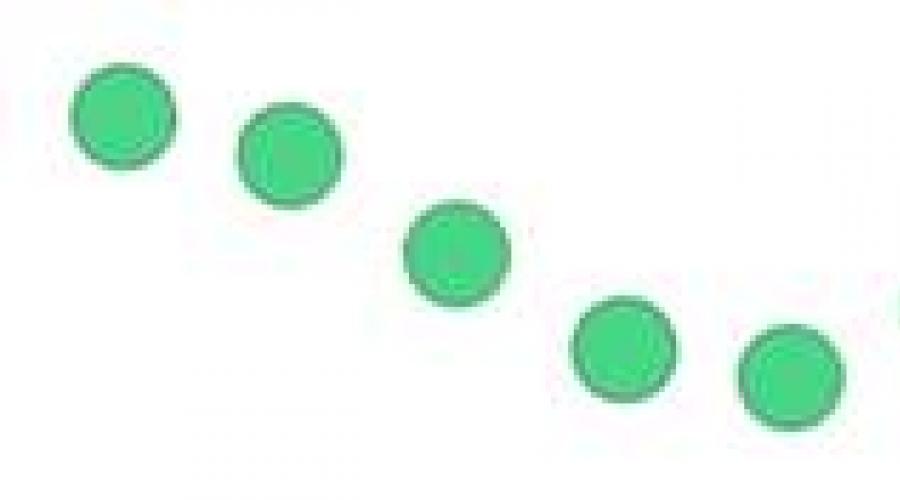
Mechanical waves
If vibrations of particles are excited in any place in a solid, liquid or gaseous medium, then due to the interaction of atoms and molecules of the medium, the vibrations begin to be transmitted from one point to another with a finite speed. The process of propagation of vibrations in a medium is called wave .
Mechanical waves there are different types. If particles of the medium in a wave are displaced in a direction perpendicular to the direction of propagation, then the wave is called transverse . An example of a wave of this kind can be waves running along a stretched rubber band (Fig. 2.6.1) or along a string.
If the displacement of particles of the medium occurs in the direction of propagation of the wave, then the wave is called longitudinal . Waves in an elastic rod (Fig. 2.6.2) or sound waves in a gas are examples of such waves.
Waves on the surface of a liquid have both transverse and longitudinal components.
In both transverse and longitudinal waves, there is no transfer of matter in the direction of wave propagation. In the process of propagation, particles of the medium only oscillate around equilibrium positions. However, waves transfer vibrational energy from one point in the medium to another.
Characteristic feature mechanical waves is that they propagate in material media (solid, liquid or gaseous). There are waves that can propagate in vacuum (for example, light waves). Mechanical waves necessarily require a medium that has the ability to store kinetic and potential energy. Therefore, the environment must have inert and elastic properties. In real environments, these properties are distributed throughout the entire volume. For example, any small element of a solid body has mass and elasticity. In the simplest one-dimensional model a solid body can be represented as a collection of balls and springs (Fig. 2.6.3).
Longitudinal mechanical waves can propagate in any media - solid, liquid and gaseous.
If in a one-dimensional model of a solid body one or more balls are displaced in a direction perpendicular to the chain, then deformation will occur shift. The springs, deformed by such a displacement, will tend to return the displaced particles to the equilibrium position. In this case, elastic forces will act on the nearest undisplaced particles, tending to deflect them from the equilibrium position. As a result, a transverse wave will run along the chain.
In liquids and gases, elastic shear deformation does not occur. If one layer of liquid or gas is displaced a certain distance relative to the adjacent layer, then no tangential forces will appear at the boundary between the layers. The forces acting at the boundary of a liquid and a solid, as well as the forces between adjacent layers of liquid, are always directed normal to the boundary - these are pressure forces. The same applies to gaseous environment. Hence, transverse waves cannot exist in liquid or gaseous media.
Of significant practical interest are simple harmonic or sine waves . They are characterized amplitudeA particle vibrations, frequencyf And wavelengthλ. Sinusoidal waves propagate in homogeneous media with some constant speed υ.
Bias y (x, t) particles of the medium from the equilibrium position in a sinusoidal wave depends on the coordinate x on the axis OX, along which the wave propagates, and on time t in law.
When vibrations of particles are excited in any place in a solid, liquid or gaseous medium, the result of the interaction of atoms and molecules of the medium is the transfer of vibrations from one point to another with a finite speed.
Definition 1
Wave is the process of propagation of vibrations in a medium.
Distinguish the following types mechanical waves:
Definition 2
Transverse wave: particles of the medium are displaced in a direction perpendicular to the direction of propagation of the mechanical wave.
Example: waves propagating along a string or rubber band in tension (Figure 2, 6, 1);
Definition 3
Longitudinal wave: particles of the medium are displaced in the direction of propagation of the mechanical wave.
Example: waves propagating in a gas or an elastic rod (Figure 2, 6, 2).
Interestingly, waves on the surface of a liquid include both transverse and longitudinal components.
Note 1
Let us point out an important clarification: when mechanical waves propagate, they transfer energy and shape, but do not transfer mass, i.e. In both types of waves, there is no transfer of matter in the direction of wave propagation. As they spread, the particles of the medium oscillate around their equilibrium positions. In this case, as we have already said, waves transfer energy, namely the energy of vibrations from one point in the medium to another.
Figure 2. 6. 1 . Propagation of a transverse wave along a rubber band in tension.
Figure 2. 6. 2. Propagation of a longitudinal wave along an elastic rod.
A characteristic feature of mechanical waves is their propagation in material media, in contrast, for example, to light waves, which can propagate in emptiness. For the occurrence of a mechanical wave impulse, a medium is required that has the ability to store kinetic and potential energy: i.e. the medium must have inert and elastic properties. In real environments, these properties are distributed throughout the entire volume. For example, every small element of a solid body has inherent mass and elasticity. The simplest one-dimensional model of such a body is a collection of balls and springs (Figure 2, 6, 3).
Figure 2. 6. 3. The simplest one-dimensional model of a solid body.
In this model, inert and elastic properties are separated. Balls have mass m, and the springs are the stiffness k. Such simple model makes it possible to describe the propagation of longitudinal and transverse mechanical waves in a solid body. When a longitudinal wave propagates, the balls are displaced along the chain, and the springs are stretched or compressed, which is a tensile or compressive deformation. If such deformation occurs in a liquid or gaseous medium, it is accompanied by compaction or rarefaction.
Note 2
A distinctive feature of longitudinal waves is that they can propagate in any media: solid, liquid and gaseous.
If in the specified model of a solid body one or more balls receive a displacement perpendicular to the entire chain, we can talk about the occurrence of shear deformation. Springs that have become deformed as a result of displacement will tend to return the displaced particles to the equilibrium position, and the nearest undisplaced particles will begin to be influenced by elastic forces tending to deflect these particles from the equilibrium position. The result will be the appearance of a transverse wave in the direction along the chain.
In a liquid or gaseous medium, elastic shear deformation does not occur. The displacement of one layer of liquid or gas by a certain distance relative to the adjacent layer will not lead to the appearance of tangential forces at the boundary between the layers. The forces that act at the boundary of a liquid and a solid, as well as the forces between adjacent layers of liquid, are always directed normal to the boundary - these are pressure forces. The same can be said about a gaseous medium.
Note 3
Thus, the appearance of transverse waves is impossible in liquid or gaseous media.
In respect of practical application Of particular interest are simple harmonic or sine waves. They are characterized by the amplitude A of particle vibrations, frequency f and wavelength λ. Sinusoidal waves propagate in homogeneous media with a certain constant speed υ.
Let us write an expression showing the dependence of the displacement y (x, t) of particles of the medium from the equilibrium position in a sine wave on the coordinate x on the O X axis along which the wave propagates, and on time t:
y (x, t) = A cos ω t - x υ = A cos ω t - k x.
In the above expression, k = ω υ is the so-called wave number, and ω = 2 π f is the circular frequency.
Figure 2. 6. 4 shows “snapshots” of a transverse wave at time t and t + Δt. Over a period of time Δt, the wave moves along the O X axis to a distance υ Δt. Such waves are called traveling waves.
Figure 2. 6. 4 . "Snapshots" of a traveling sine wave at a moment in time t and t + Δt.
Definition 4
Wavelengthλ is the distance between two adjacent points on the axis O X oscillating in the same phases.
The distance, the value of which is the wavelength λ, the wave travels during the period T. Thus, the wavelength formula has the form: λ = υ T, where υ is the speed of propagation of the wave.
Over time t, the coordinate changes x of any point on the graph displaying the wave process (for example, point A in Figure 2. 6. 4), while the value of the expression ω t – k x remains unchanged. After time Δt, point A will move along the axis O X to some distance Δ x = υ Δ t . Thus:
ω t - k x = ω (t + ∆ t) - k (x + ∆ x) = c o n s t or ω ∆ t = k ∆ x.
From this expression it follows:
υ = ∆ x ∆ t = ω k or k = 2 π λ = ω υ .
It becomes obvious that a traveling sine wave has a double periodicity - in time and space. The time period is equal to the oscillation period T of the particles of the medium, and the spatial period equal to length waves λ.
Definition 5
Wave number k = 2 π λ is a spatial analogue of the circular frequency ω = - 2 π T .
Let us emphasize that the equation y (x, t) = A cos ω t + k x is a description of a sine wave propagating in the direction opposite to the direction of the axis O X, with speed υ = - ω k.
When a traveling wave propagates, all particles of the medium oscillate harmoniously with a certain frequency ω. This means that, as with a simple oscillatory process, the average potential energy, which is the reserve of a certain volume of the medium, is the average kinetic energy in the same volume, proportional to the square of the oscillation amplitude.
Note 4
From the above we can conclude that when a traveling wave propagates, an energy flow appears proportional to the speed of the wave and the square of its amplitude.
Traveling waves move in a medium at certain speeds, depending on the type of wave, inert and elastic properties of the medium.
The speed with which transverse waves propagate in a stretched string or rubber band depends on the linear mass μ (or mass per unit length) and the tension force T:
The speed with which longitudinal waves propagate in an unbounded medium is calculated with the participation of such quantities as the density of the medium ρ (or mass per unit volume) and the modulus of compression B (equal to the coefficient proportionality between the change in pressure Δ p and the relative change in volume Δ V V taken with the opposite sign):
∆ p = - B ∆ V V .
Thus, the speed of propagation of longitudinal waves in an infinite medium is determined by the formula:
Example 1
At a temperature of 20 ° C, the speed of propagation of longitudinal waves in water is υ ≈ 1480 m/s, in different varieties steel υ ≈ 5 – 6 k m/s.
If we're talking about about longitudinal waves propagating in elastic rods, the formula for the wave speed contains not the modulus of uniform compression, but the Young’s modulus:
For steel the difference E from B insignificant, but for other materials it can be 20–30% or more.
Figure 2. 6. 5 . Model of longitudinal and transverse waves.
Suppose that a mechanical wave, which has spread in a certain medium, encounters some obstacle on its way: in this case, the nature of its behavior will change dramatically. For example, at the interface between two media with different mechanical properties the wave will be partially reflected and partially penetrated into the second medium. A wave running along a rubber band or string will be reflected from the fixed end, and a counter wave will appear. If both ends of the string are fixed, complex vibrations will appear, which are the result of the superposition (superposition) of two waves propagating in opposite directions and experiencing reflections and re-reflections at the ends. This is how the strings of all strings “work” musical instruments, fixed at both ends. A similar process occurs with the sound of wind instruments, in particular organ pipes.
If waves propagating along a string in counter directions have a sinusoidal shape, then under certain conditions they form a standing wave.
Suppose a string of length l is fixed in such a way that one of its ends is located at point x = 0, and the other at point x 1 = L (Figure 2. 6. 6). There is tension in the string T.
Drawing 2 . 6 . 6 . The appearance of a standing wave in a string fixed at both ends.
Two waves with the same frequency simultaneously run along the string in opposite directions:
- y 1 (x , t) = A cos (ω t + k x) – wave propagating from right to left;
- y 2 (x, t) = A cos (ω t - k x) – a wave propagating from left to right.
Point x = 0 is one of the fixed ends of the string: at this point the incident wave y 1 as a result of reflection creates a wave y 2. Reflecting from the fixed end, the reflected wave enters into antiphase with the incident one. In accordance with the principle of superposition (which is an experimental fact), the vibrations created by counter-propagating waves at all points of the string are summed up. From the above it follows that the final oscillation at each point is determined as the sum of oscillations caused by waves y 1 and y 2 separately. Thus:
y = y 1 (x, t) + y 2 (x, t) = (- 2 A sin ω t) sin k x.
The given expression is a description of a standing wave. Let us introduce some concepts applicable to such a phenomenon as a standing wave.
Definition 6
Nodes– points of immobility in a standing wave.
Antinodes– points located between nodes and oscillating with maximum amplitude.
If we follow these definitions, for a standing wave to occur, both fixed ends of the string must be nodes. The formula stated earlier meets this condition at the left end (x = 0). For the condition to be satisfied at the right end (x = L), it is necessary that k L = n π, where n is any integer. From the above we can conclude that a standing wave in a string does not always appear, but only when the length L string is equal to an integer number of half-wave lengths:
l = n λ n 2 or λ n = 2 l n (n = 1, 2, 3, ...) .
A set of wavelength values λ n corresponds to a set of possible frequencies f
f n = υ λ n = n υ 2 l = n f 1 .
In this notation, υ = T μ is the speed with which transverse waves propagate along the string.
Definition 7
Each of the frequencies f n and the associated type of string vibration is called a normal mode. The smallest frequency f 1 is called the fundamental frequency, all others (f 2, f 3, ...) are called harmonics.
Figure 2. 6. Figure 6 illustrates the normal mode for n = 2.
A standing wave has no energy flow. The vibration energy “locked” in a section of string between two adjacent nodes is not transferred to the rest of the string. In each such segment there is a periodic (twice per period) T) transformation kinetic energy into potential and back, like an ordinary oscillatory system. However, there is a difference here: if a load on a spring or a pendulum has a single natural frequency f 0 = ω 0 2 π, then the string is characterized by the presence of an infinite number of natural (resonant) frequencies f n. In Figure 2. 6. Figure 7 shows several variants of standing waves in a string fixed at both ends.
Figure 2. 6. 7. The first five normal modes of vibration of a string fixed at both ends.
According to the superposition principle, standing waves various types(With different meanings n) are capable of simultaneously being present in the vibrations of the string.
Figure 2. 6. 8 . Model of normal modes of a string.
If you notice an error in the text, please highlight it and press Ctrl+Enter
Wave process- the process of transferring energy without transferring matter.
Mechanical wave- a disturbance propagating in an elastic medium.
The presence of an elastic medium is a necessary condition for the propagation of mechanical waves.
The transfer of energy and momentum in a medium occurs as a result of interaction between neighboring particles of the medium.
Waves are longitudinal and transverse.
Longitudinal mechanical wave is a wave in which the movement of particles of the medium occurs in the direction of propagation of the wave. A transverse mechanical wave is a wave in which particles of the medium move perpendicular to the direction of propagation of the wave.
Longitudinal waves can propagate in any medium. Transverse waves do not arise in gases and liquids, since in them
there are no fixed positions of particles.
Periodic external influence causes periodic waves.
Harmonic wave- a wave generated by harmonic vibrations of particles of the medium.
Wavelength- the distance over which the wave propagates during the period of oscillation of its source:
Mechanical wave speed- speed of propagation of disturbance in the medium. Polarization is the ordering of the directions of vibrations of particles in a medium.
Plane of polarization- the plane in which particles of the medium vibrate in a wave. A linearly polarized mechanical wave is a wave whose particles oscillate along a certain direction (line).
Polarizer- a device that emits a wave of a certain polarization.
standing wave- a wave formed as a result of the superposition of two harmonic waves propagating towards each other and having the same period, amplitude and polarization.
Standing wave antinodes- position of points with maximum amplitude of oscillations.
Standing wave nodes- non-moving wave points whose oscillation amplitude is zero.
Along the length l of the string, fixed at the ends, an integer n half-waves of transverse standing waves fit:

Such waves are called oscillation modes.
The mode of oscillation for an arbitrary integer n > 1 is called nth harmonic or nth overtone. The mode of vibration for n = 1 is called the first harmonic or fundamental mode of vibration. Sound waves are elastic waves in a medium that cause auditory sensations in humans.
The frequency of vibrations corresponding to sound waves ranges from 16 Hz to 20 kHz.
The speed of propagation of sound waves is determined by the speed of transmission of interactions between particles. The speed of sound in a solid vp is, as a rule, greater than the speed of sound in a liquid vg, which, in turn, exceeds the speed of sound in a gas vg.

Sound signals are classified by pitch, timbre and volume. The pitch of the sound is determined by the frequency of the source sound vibrations. The higher the vibration frequency, the higher the sound; vibrations of low frequencies correspond to low sounds. The timbre of a sound is determined by the shape of sound vibrations. The difference in the shape of vibrations having the same period is associated with different relative amplitudes of the fundamental mode and overtone. The loudness of a sound is characterized by the level of intensity of the sound. Sound intensity is the energy of sound waves incident on an area of 1 m2 in 1 s.
Topics of the Unified State Examination codifier: mechanical waves, wavelength, sound.
Mechanical waves is the process of propagation of vibrations of particles of an elastic medium (solid, liquid or gaseous) in space.
The presence of elastic properties in a medium is a necessary condition wave propagation: deformation that occurs in any place, due to the interaction of neighboring particles, is sequentially transmitted from one point in the medium to another. Various types deformations will correspond different types waves
Longitudinal and transverse waves.
The wave is called longitudinal, if the particles of the medium oscillate parallel to the direction of propagation of the wave. A longitudinal wave consists of alternating tensile and compressive deformations. In Fig.
Figure 1 shows a longitudinal wave, which represents vibrations of flat layers of the medium; the direction along which the layers oscillate coincides with the direction of wave propagation (i.e., perpendicular to the layers).
A wave is called transverse if the particles of the medium oscillate perpendicular to the direction of propagation of the wave. A transverse wave is caused by shear deformations of one layer of the medium relative to another. In Fig. 2, each layer oscillates along itself, and the wave goes perpendicular to the layers. Longitudinal waves can propagate in
solids
, liquids and gases: in all these media an elastic reaction to compression occurs, as a result of which compression and rarefaction of the medium will appear one after another.
However, liquids and gases, unlike solids, do not have elasticity with respect to the shear of layers. Therefore, transverse waves can propagate in solids, but not inside liquids and gases*..
It is important to note that the particles of the medium, when a wave passes, oscillate near unchanged equilibrium positions, i.e., on average, they remain in their places. The wave thus carries out transfer of energy not accompanied by transfer of matter Easiest to learn harmonic waves. They are caused by external influences on the environment, changing according to a harmonic law. When a harmonic wave propagates, the particles of the medium perform harmonic vibrations external influence. In what follows we will limit ourselves to harmonic waves.
Let us consider the process of wave propagation in more detail. Let us assume that some particle of the medium (particle) began to oscillate with a period. Acting on a neighboring particle, it will pull it along with it. The particle, in turn, will pull the particle along with it, etc. This will create a wave in which all particles will oscillate with a period.
However, particles have mass, that is, they are inert. It takes some time for their speed to change. Consequently, the particle in its movement will lag somewhat behind the particle, the particle will lag behind the particle, etc. When the particle has completed its first oscillation and begins the second, a particle located at a certain distance from the particle will begin its first oscillation.
So, in a time equal to the period of particle oscillations, the disturbance of the medium propagates over a distance. This distance is called wavelength. The oscillations of a particle will be identical to the oscillations of a particle, the oscillations of the next particle will be identical to the oscillations of a particle, etc. The oscillations, as it were, reproduce themselves at a distance, we can call spatial period of oscillation; along with the time period it is the most important characteristic wave process. In a longitudinal wave, the wavelength is equal to the distance between adjacent compressions or rarefactions (Fig. 1). Transversely - the distance between adjacent humps or depressions (Fig. 2). In general, the wavelength is equal to the distance (along the direction of propagation of the wave) between two nearest particles of the medium that oscillate equally (that is, with a phase difference equal to ).
Wave propagation speed is called the ratio of wavelength to the period of oscillation of particles of the medium:
The frequency of a wave is the frequency of particle oscillations:
From here we get the relationship between wave speed, wavelength and frequency:
. (1)
Sound.
Sound waves V in a broad sense are called all kinds of waves propagating in an elastic medium. In a narrow sense sound are sound waves in the frequency range from 16 Hz to 20 kHz, perceived by the human ear. Below this range lies the area infrasound, above - area ultrasound.
The main characteristics of sound include volume And height.
The loudness of a sound is determined by the amplitude of pressure fluctuations in sound wave and is measured in special units - decibels(dB). Thus, a volume of 0 dB is the threshold of audibility, 10 dB is the ticking of a clock, 50 dB is a normal conversation, 80 dB is a scream, 130 dB is the upper limit of audibility (the so-called pain threshold).
Tone is the sound produced by a body performing harmonic vibrations (for example, a tuning fork or a string). The pitch of a tone is determined by the frequency of these vibrations: the higher the frequency, the higher the sound seems to us. So, by tightening the string, we increase the frequency of its vibrations and, accordingly, the pitch of the sound.
The speed of sound in different media is different: the more elastic the medium is, the faster sound travels through it. In liquids the speed of sound is greater than in gases, and in solids it is greater than in liquids.
For example, the speed of sound in air is approximately 340 m/s (it is convenient to remember it as “a third of a kilometer per second”)*. In water, sound travels at a speed of about 1500 m/s, and in steel - about 5000 m/s.
notice, that frequency sound from a given source in all media is the same: particles of the medium perform forced oscillations with the frequency of the sound source. According to formula (1), we then conclude that when moving from one medium to another, along with the speed of sound, the length of the sound wave changes.