Physicochemical methods for assessing composition and structure. Acoustic methods for studying building materials
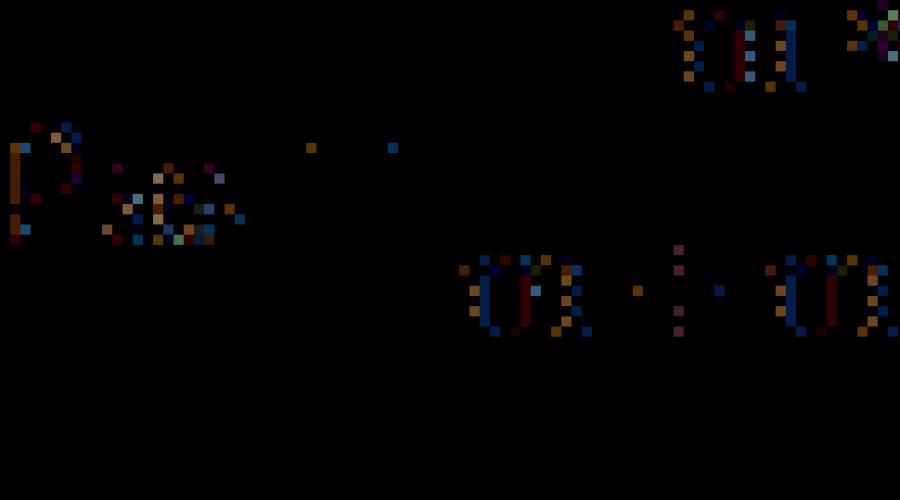
Read also
Goal of the work: 1. Familiarize yourself with the basic methods for studying the properties of building materials.
2. Analyze the basic properties of building materials.
1. Determination of the true (absolute) density of the material
(pycnometric method) (GOST 8269)
To determine the true density, crushed building materials are taken: brick, limestone crushed stone, expanded clay gravel, they are crushed, passed through a sieve with a mesh of less than 0.1 mm, and a sample weighing 10 g each (m) is taken.
Each sample is poured into a clean, dried pycnometer (Fig. 1) and distilled water is poured into it in such an amount that the pycnometer is filled to no more than half its volume, then the pycnometer is shaken, wetting all the powder, placed in a sand bath and the contents are heated. until boiling in an inclined position for 15-20 minutes to remove air bubbles.
Rice. 1 – Pycnometer for determining the true density of the material
Then the pycnometer is wiped, cooled to room temperature, add distilled water to the mark and weigh (m 1), after which the pycnometer is emptied of its contents, washed, filled to the mark with distilled water at room temperature and weighed again (m 2). A table is drawn in the notebook in which the masses of each material and subsequent calculations are entered.
The true density of the material is determined by the formula:
where is the mass of the powder sample, g;
Weight of pycnometer with sample and water after boiling, g;
Weight of pycnometer with water, g;
The density of water is 1 g/cm3.
2. Determination of the correct average density of the sample geometric shape(GOST 6427)
It is better to determine the average density from the same materials - bricks, a piece of limestone and expanded clay gravel. The volume of samples of regular geometric shape (brick) is determined by geometric dimensions in accordance with the drawing, measured with an error of no more than 0.1 mm. Each linear dimension is calculated as the arithmetic mean of three measurements. Samples must be dry.
The volume of irregularly shaped samples is determined from the displaced water by dropping a piece of limestone or gravel that sinks into a graduated cylinder of water, with a mark on the volume of displaced fluid. 1ml=1cm 3.
Rice. 1 – Measurement of linear dimensions and volume of a sample
prisms cylinder
Average density determined by the formula:
where is the mass of the dry sample, g;
Sample volume, cm3.
No. | Material | P, % | ||||||||
brick | ||||||||||
limestone | ||||||||||
expanded clay | ||||||||||
sq. sand | ||||||||||
3. Determination of material porosity (GOST 12730.4)
Knowing the true density and average density brick, limestone, expanded clay gravel, determine the porosity of the material P,%, according to the formula:
where is the average density of the material, g/cm 3 or kg/m 3 ;
True density of the material, g/cm3 or kg/m3.
Comparative Density different materials is given in Appendix A. The results are entered into the table.
4. Determination of bulk density (GOST 8269)
Bulk material (sand, expanded clay gravel, crushed stone) in a volume sufficient to carry out the test is dried to a constant weight. The material is poured into a pre-weighed measuring cylinder (m) from a height of 10 cm until a cone is formed, which is removed with a steel ruler flush with the edges (without compaction) moving towards you, after which the cylinder with the sample is weighed (m 1).
Rice. 3. Funnel for determining the bulk density of sand
1 – funnel; 2 – supports; 3 – damper
Bulk density of material determined by the formula:
where is the mass graduated cylinder, G;
Mass of measuring cylinder with attachment, g;
Volume of graduated cylinder, l.
The results are entered into the table.
5. Determination of voidness (GOST 8269)
Voidness (V empty, %) bulk material determined by knowing the bulk and average density of the bulk material using the formula:
where is the bulk density of the material, kg/m3;
Average density of the material, kg/m3.
The average density of quartz sand is not determined; it is accepted as true - 2.65 g/cm 3 .
6. Determination of material moisture (GOST 8269)
A sample of the material in the amount of 1.5 kg is poured into a vessel and weighed, then dried to a constant weight in drying cabinet(this must be done in advance). To determine humidity in a lesson, you can do the opposite: weigh an arbitrary amount of dry sand in a vessel and wet it arbitrarily, weigh it again, getting and.
Humidity W,%, is determined by the formula:
where is the mass of the wet sample, g;
Dry mass of sample, g.
To determine water absorption, three samples of any shape measuring from 40 to 70 mm or a brick are taken and the volume is determined. Clean the samples from dust with a wire brush and dry to a constant weight. Then they are weighed and placed in a vessel with water at room temperature so that the water level in the vessel is at least 20 mm above the top of the samples. The samples are kept in this position for 48 hours. After which they are taken out of the water, moisture is removed from the surface with a wrung-out damp soft cloth, and each sample is weighed.
Water absorption by mass Wab,%, is determined by the formula:
Water absorption by volume W o,%, is determined by the formula:
where is the dry mass of the sample, g;
Mass of the sample after saturation with water, g;
Volume of the sample in its natural state, cm3.
Relative density is defined as:
The coefficient of saturation of the material with water is determined:
Having calculated all the indicators with the teacher, the student receives individual task according to the variants of problems in test No. 1.
7. Determination of compressive strength (GOST 8462)
Compressive strength is determined on cubes of sizes 7.07 × 7.07 × 7.07 cm, 10 × 10 × 10 cm, 15 × 15 × 15 cm and 20 × 20 × 20 cm. Bricks and beams are first tested for bending strength (8), then the halves are tested in compression.
To determine compressive strength, samples of regular geometric shape (beams, cubes, bricks) are inspected, measured and tested on a hydraulic press. Place the sample in the center of the base plate and press it with the top plate of the press, which should fit tightly along the entire edge of the sample. During testing, the load on the sample must increase continuously and evenly. The highest compressive load corresponds to the maximum pressure gauge reading during the test.
When testing the compressive strength of cubes, the top face of the cube should become the side face to eliminate unevenness.
The compressive strength R compressed, MPa, for concrete cube samples is determined by the formula:
where is the maximum breaking load, kN;
Square cross section sample (arithmetic mean of the areas of the upper and lower faces), cm 2.
8. Determination of bending strength. (GOST 8462)
The tensile strength in bending is determined on samples - beams using universal machine MII-100, which immediately gives readings etc. weight in kg/cm 2 or on brick using a hydraulic press using rollers according to the scheme proposed in Figure 5. Tests of the strength of the brick must be shown, then the compressive strength of the halves (9) and the brand of brick must be determined.
Rice. 4 – MII-100 testing machine for determining bending strength
Fig. 5 – Scheme of bending strength test
Ultimate bending strength R bend, MPa, is determined by the following formula:
Distance between support axes, cm;
Sample width, cm;
Sample height, cm.
Material | |||||||
brick | |||||||
beam | |||||||
cube |
9. Determination of the coefficient of structural quality (specific strength of the material)
Enter the calculation results into the table.
1. What are the main properties of building materials, which ones are important for structural materials?
2. What densities are determined for building materials, and how?
3. What is true density? Why is it defined?
4. What is bulk density? How is it determined and why?
5. To determine the average density, what volume do you need to know? How to determine the volume of a piece of crushed stone?
6. Which density has the greatest numeric expression for the same material, which is the smallest? Why?
7. For what materials is voidness determined, how does it differ from porosity? Compare the true, average, and bulk densities of quartz sand, brick, expanded clay gravel or crushed limestone.
8. What is the relationship between total porosity and density? What is porosity?
9. What porosity can the material have? How can it be determined?
10. Does porosity affect the moisture content of a material? What is humidity?
11. How does humidity differ from water absorption? What properties can be judged by knowing water absorption?
12. How to determine the water saturation coefficient? What does it characterize?
13. How to determine the softening coefficient? What is its significance for air and hydraulic binders?
14. Will water and gas permeability change with a change in density, how? At what type of porosity do these indicators increase?
15. Does the amount of porosity affect the amount of swelling and shrinkage of the material? What is the shrinkage of cellular concrete, what is heavy concrete?
16. Is there a connection between the density of a material and thermal conductivity? What materials protect better from the cold? What density material are the walls of residential buildings made from?
17. Does moistening the material affect the thermal conductivity coefficient? Why?
18. What is the coefficient of linear thermal expansion for concrete, steel, granite, wood? When does it matter?
19. Is it possible to use materials with Kn = 1 for the manufacture of slabs road surface? Why?
20. How does porosity differ from voidness, and what formula is used to determine these indicators?
21. Are there materials whose true density is equal to the average?
22. Why do pores form in bricks? Does the method of brick molding affect their number?
23. How do we increase porosity in artificial stone, why?
24. What causes shrinkage, which materials have it more: dense or porous?
25. Does shrinkage depend on the water absorption of the material? What water in the structure of the material does not evaporate?
26. On what samples is the strength of binders, mortars and concrete determined, by what formula is the strength calculated, in what units?
27. On what indicators does strength depend, and in which structures is it maximum?
28. Why do some materials have greater flexural strength, while others have less compressive strength? What are such materials called?
29. What characteristics does frost resistance depend on?
30. What is called specific surface area? Does moisture depend on this characteristic?
Laboratory work No. 4
Gypsum binders
Goal of the work: 1. Familiarize yourself with the basic properties of building gypsum.
2. Analyze the main properties of building gypsum.
IntroductionThroughout its development, humanity uses the laws of chemistry and physics in its activities to solve various problems and satisfy many needs.
In ancient times, this process took place in two different ways: consciously, based on accumulated experience, or by chance. TO striking examples conscious application of the laws of chemistry include: souring of milk, and its subsequent use for the preparation of cheese products, sour cream and other things; fermentation of certain seeds, for example, hops and subsequent production of brewing products; fermentation of the juices of various fruits (mainly grapes, which contain a large amount of sugar), ultimately produced wine products and vinegar.
The discovery of fire was a revolution in the life of mankind. People began to use fire to cook food, heat treatment clay products, for working with various metals, for obtaining charcoal and much more.
Over time, people have developed a need for more functional materials and products based on them. Their knowledge in the field of chemistry had a huge impact on solving this problem. Chemistry played a particularly important role in the production of pure and ultrapure substances. If in the manufacture of new materials, the first place belongs to physical processes and technologies based on them, then the synthesis of ultrapure substances, as a rule, is more easily accomplished using chemical reactions [
Using physicochemical methods, they study the physical phenomena that arise during chemical reactions. For example, in the colorimetric method, the color intensity is measured depending on the concentration of the substance; in the conductometric method, the change in the electrical conductivity of solutions is measured; optical methods use the relationship between the optical properties of the system and its composition.
Physico-chemical methods The research is also used for a comprehensive study of building materials. The use of such methods allows for an in-depth study of the composition, structure and properties of building materials and products. Diagnostics of the composition, structure and properties of the material on different stages its production and operation makes it possible to develop progressive resource-saving and Energy Saving Technologies [
The above work shows the general classification of physicochemical methods for studying building materials (thermography, radiography, optical microscopy, electron microscopy, atomic emission spectroscopy, molecular absorption spectroscopy, colorimetry, potentiometry) and methods such as thermal and X-ray phase analysis, as well as methods for studying porous structure [ Builder's Handbook [ Electronic resource] // Ministry of Urban and Rural Construction of the Belarusian SSR. URL: www.bibliotekar.ru/spravochnick-104-stroymaterialy.html].
1. Classification of physical and chemical research methods
Physico-chemical research methods are based on the close connection between the physical characteristics of the material (for example, the ability to absorb light, electrical conductivity, etc.) and the structural organization of the material from a chemical point of view. It happens that from physicochemical methods, purely physical research methods are distinguished as a separate group, thus showing that physicochemical methods consider a certain chemical reaction, in contrast to purely physical ones. These research methods are often called instrumental because they involve the use of various measuring devices. Instrumental research methods, as a rule, have their own theoretical base; this base differs from the theoretical base of chemical research (titrimetric and gravimetric). It was based on the interaction of matter with various energies.
In the course of physical and chemical research, in order to obtain the necessary data on the composition and structural organization of a substance, an experimental sample is exposed to the influence of some kind of energy. Depending on the type of energy in a substance, the energy states of its constituent particles (molecules, ions, atoms) change. This is expressed in a change in a certain set of characteristics (for example, color, magnetic properties and others). As a result of recording changes in the characteristics of a substance, data is obtained on the qualitative and quantitative composition of the test sample, or data on its structure.
According to the type of influencing energies and characteristics being studied, physico-chemical research methods are divided in the following way.
Table 1. Classification of physicochemical methods
In addition to those given in this table, there are quite a lot of private physicochemical methods that do not fit this classification. In fact, the most actively used are optical, chromatographic and potentiometric methods for studying the characteristics, composition and structure of a sample [ Galuso, G.S. Methods for studying building materials: teaching aid/ G.S. Galuzo, V.A. Bogdan, O.G. Galuzo, V.I. Kovazhnkova. – Minsk: BNTU, 2008. – 227 p.].
2. Thermal analysis methods
Thermal analysis is actively used to study various building materials - mineral and organic, natural and synthetic. Its use helps to identify the presence of a particular phase in a material, determine interaction reactions, decomposition and, in exceptional cases, obtain information about the quantitative composition of the crystalline phase. The ability to obtain information on the phase composition of highly dispersed and cryptocrystalline polymineral mixtures without dividing into polymineral fractions is one of the main advantages of the technique. Thermal research methods are based on the rules of constancy chemical composition And physical characteristics substances, in specific conditions, and, among other things, on the laws of correspondence and characteristicality.
The correspondence law says that a specific thermal effect can be correlated with any phase change in a sample.
And the law of characteristicality states that thermal effects are individual for each chemical substance.
The main idea of thermal analysis is to study the transformations that occur under conditions of increasing temperature in systems of substances or specific compounds during various physical and chemical processes, according to the thermal effects that accompany them.
Physical processes, as a rule, are based on the transformation of the structural structure, or state of aggregation system with its constant chemical composition.
Chemical processes lead to a transformation of the chemical composition of the system. These include directly dehydration, dissociation, oxidation, exchange reactions and others.
Initially, thermal curves for limestone and clayey rocks were obtained by the French chemist Henri Louis Le Chatelier in 1886 - 1887. In Russia, academician N.S. was one of the first to study thermal research methods. Kurnakov (in 1904). Updated modifications of the Kurnakov pyrometer (device for automatic recording heating and cooling curves) are still used in most research laboratories today. Regarding the characteristics under study as a result of heating or cooling, the following methods of thermal analysis are distinguished: differential thermal analysis (DTA) - the change in energy of the sample under study is determined; thermogravimetry – mass changes; dilatometry – volumes change; gas volumetry – the composition of the gas phase changes; electrical conductivity – electrical resistance changes.
During thermal research, several study methods can be used in parallel, each of which records changes in energy, mass, volume and other characteristics. A comprehensive study of the characteristics of the system during the heating process helps to study in more detail and more thoroughly the basics of the processes occurring in it.
One of the most important and widely used methods is differential thermal analysis.
Fluctuations in the temperature characteristics of a substance can be detected by sequentially heating it. So, the crucible is filled with experimental material (breakdown), placed in an electric furnace, which heats up, and the temperature readings of the system under study begin to be taken using a simple thermocouple connected to a galvanometer.
Registration of changes in the enthalpy of a substance occurs using an ordinary thermocouple. But due to the fact that the deviations that can be seen on the temperature curve are not very large, it is better to use a differential thermocouple. Initially, the use of this thermocouple was proposed by N.S. Kurnakov. A schematic representation of a self-registering pyrometer is presented in Figure 1.
This schematic image shows a pair of ordinary thermocouples, which are connected to each other by the same ends, forming the so-called cold junction. The remaining two ends are connected to the apparatus, which makes it possible to record transformations in the electromotive force (EMF) circuit that appear as a result of an increase in the temperature of the hot junctions of the thermocouple. One hot junction is located in the sample being studied, and the second is located in the reference substance.
Figure 1. Schematic representation of a differential and simple thermocouple: 1 – electric furnace; 2 – block; 3 – experimental sample under study; 4 – reference substance (standard); 5 – thermocouple hot junction; 6 – cold junction of thermocouple; 7 – galvanometer for fixing the DTA curve; 8 – galvanometer for recording the temperature curve.
If, for the system under study, some transformations that are associated with the absorption or release of thermal energy are frequent, then its temperature indicator at a given moment may be much higher or lower compared to the reference substance of comparison. This temperature difference leads to a difference in the EMF value and, as a consequence, to a deviation of the DTA curve up or down from zero or the base line. The zero line is the line parallel to the x-axis and drawn through the beginning of the DTA curve; this can be seen in Figure 2.
Figure 2. Schematic of simple and differential (DTA) temperature curves.
In fact, often after the completion of some thermal transformation, the DTA curve does not return to the zero line, but continues to run parallel to it or at a certain angle. This line is called the baseline. This discrepancy between the base and zero lines is explained by different thermophysical characteristics of the studied system of substances and the reference substance of comparison [].
3. X-ray phase analysis methods
X-ray methods for studying building materials are based on experiments in which X-rays are used. This class of research is actively used to study the mineralogical composition of raw materials and final products, phase transformations in the substance at various stages of their processing into ready-to-use products and during operation, and, among other things, to identify the nature of the structural structure of the crystal lattice.
The X-ray diffraction technique used to determine the parameters of the unit cell of a substance is called the X-ray diffraction technique. The technique that is followed in the study of phase transformations and the mineralogical composition of substances is called X-ray phase analysis. X-ray phase analysis (XRF) methods have great importance when studying mineral building materials. Based on the results of X-ray phase studies, information is obtained about the presence of crystalline phases and their quantities in the sample. It follows from this that there are quantitative and qualitative methods of analysis.
The purpose of qualitative X-ray phase analysis is to obtain information about the nature of the crystalline phase of the substance being studied. The methods are based on the fact that each specific crystalline material has a specific x-ray pattern with its own set of diffraction maxima. Nowadays, there is reliable radiographic data on most known to man crystalline substances.
The task of quantitative composition is to obtain information about the amount of specific phases in polyphase polycrystalline substances; it is based on the dependence of the intensity of diffraction maxima on percentage phase under study. As the amount of any phase increases, its reflection intensity becomes greater. But for polyphase substances, the relationship between the intensity and quantity of this phase is ambiguous, since the magnitude of the reflection intensity of a given phase depends not only on its percentage content, but also on the value of μ, which characterizes how much the x-ray beam is attenuated as a result of passing through the material under study . This attenuation value of the material being studied depends on the attenuation values and the number of other phases that are also included in its composition. It follows from this that each quantitative analysis technique must somehow take into account the effect of the attenuation index as a result of changes in the composition of the samples, which violates the direct proportionality between the amount of this phase and the intensity of its diffraction reflection [ Makarova, I.A. Physico-chemical methods for studying building materials: tutorial/ I.A. Makarova, N.A. Lokhova. – Bratsk: Iz-vo BrGU, 2011. – 139 p. ].
Options for obtaining X-ray images are divided, based on the method of recording radiation, into photographic and diffractometric. The use of methods of the first type involves photographic recording of X-ray radiation, under the influence of which darkening of the photographic emulsion is observed. Diffractometric methods for obtaining X-ray patterns, which are implemented in diffractometers, differ from photographic methods in that the diffraction pattern is obtained sequentially over time [ Pindyuk, T.F. Methods for studying building materials: guidelines for laboratory work / T.F. Pindyuk, I.L. Chulkova. – Omsk: SibADI, 2011. – 60 p. ].
4. Methods for studying porous structure
Building materials have a heterogeneous and rather complex structure. Despite the variety and origin of materials (concrete, silicate materials, ceramics) there are always various pores in their structure.
The term “porosity” connects the two most important properties of a material – geometry and structure. Geometric characteristic, this is the total pore volume, pore size and their total specific surface area, which determine the porosity of the structure (large-porous material or fine-porous one). Structural characteristics– this is the type of pores and their distribution by size. These properties vary depending on the structure of the solid phase (granular, cellular, fibrous, etc.) and the structure of the pores themselves (open, closed, communicating).
The main influence on the size and structure of porous formations is exerted by the properties of the feedstock, the composition of the mixture, and the production process. The most important characteristics are the particle size distribution, binder volume, percentage of moisture in the feedstock, methods of forming the final product, conditions for the formation of the final structure (sintering, fusion, hydration, etc.). Specialized additives, so-called modifiers, have a strong influence on the structure of porous formations. These include, for example, fuel and burn-out additives, which are added to the charge during the production of ceramic products, and in addition to surfactants, they are used both in ceramics and in cement-based materials. The pores differ not only in size, but also in shape, and the capillary channels they create have a variable cross-section along their entire length. All pore formations are classified into closed and open, as well as channel-forming and dead-end.
The structure of porous building materials is characterized by the combination of all types of pores. Porous formations can be randomly located inside a substance, or they can have some order.
Pore channels have a very complex structure. Closed pores are cut off from open pores and are in no way connected with each other or with the external environment. This class of pores is impermeable to gaseous substances and liquids and, as a result, is not considered hazardous. Open channel-forming and dead-end porous formations water environment can be filled in without difficulty. Their filling proceeds through various schemes and depends mainly on the cross-sectional area and length of the pore channels. As a result of ordinary saturation, not all porous channels can be filled with water; for example, the smallest pores less than 0.12 microns in size are never filled due to the presence of air in them. Large porous formations fill very quickly, but air environment, as a result of the low value of capillary forces, water is poorly retained in them.
The volume of water absorbed by a substance depends on the size of the porous formations and on the adsorption characteristics of the material itself.
To determine the relationship between the porous structure and physical and chemical characteristics It is not enough for the material to know only the general value of the volume of porous formations. General porosity does not determine the structure of a substance; the principle of pore size distribution and the presence of porous formations of a specific size play an important role here.
Geometric and structural indicators of porosity of building materials differ both at the micro and macro levels. G.I. Gorchakov and E.G. Muradov developed an experimental and computational technique for identifying total and group porosity concrete materials. The basis of the methodology is that during the experiment the level of hydration of cement in concrete is determined using quantitative X-ray examination or approximately by the volume of water ω bound in the cement binder that did not evaporate during drying at a temperature of 150 ºC: α = ω/ ω max .
The volume of bound water with complete hydration of cement is in the range of 0.25 – 0.30 (to the mass of uncalcined cement).
Then, using the formulas from Table 1, the porosity of concrete is calculated depending on the level of cement hydration, its consumption in concrete and the amount of water [ Makarova, I.A. Physico-chemical methods for studying building materials: textbook / I.A. Makarova, N.A. Lokhova. – Bratsk: Iz-vo BrGU, 2011. – 139 p. ].
Ministry of Education of the Kyrgyz Republic
Ministry of Education of the Russian Federation
Kyrgyz-Russian Slavic University
Faculty of Architecture Design and Construction
Essay
On the topic :
“The role of physical and chemical research methods in building materials”
Completed by: Mikhail Podyachev gr. PGS 2-07
Checked by: Dzhekisheva S.D.
Plan
1. Introduction…………………………………………………………………….……p. 3
2 . Physico-chemical methods of analysis and their classification………………….p. 3-83. Basic building materials studied by physics chemical methods….page 8-94. Characteristics of corrosion processes in building materials…. pp. 9-13
5. Physico-chemical methods for studying corrosion in building materials………………p. 13-15
6. Methods for protecting building materials from corrosion………………………p. 15
7. Results of corrosion research based on physicochemical methods………p. 16-18
8. Innovative methods Corrosion studies…………………………p. 18-20
9. Conclusion……………………………………………………………………p. 20
10. References……………………………………………………………page 21
Introduction.
Throughout its development, human civilization, at least in the material sphere, constantly uses chemical, biological and physical laws operating on our planet to satisfy one or another of its needs.
In ancient times, this happened in two ways: consciously or spontaneously. Naturally, we are interested in the first way. An example of the conscious use of chemical phenomena can be:
-
souring of milk, used to produce cheese, sour cream and other dairy products;
-
fermentation of certain seeds, such as hops, in the presence of yeast to form beer;
-
sublimation of pollen of some flowers (poppy, hemp) and obtaining drugs;
-
fermentation of the juice of certain fruits (primarily grapes), containing a lot of sugar, resulting in wine and vinegar.
Fire brought revolutionary changes in human life. Man began to use fire for cooking, in pottery production, for processing and smelting metals, processing wood into coal, evaporating and drying food for the winter.
Over time, people began to need more and more new materials. Chemistry provided invaluable assistance in their creation. The role of chemistry is especially great in the creation of pure and ultrapure materials (hereinafter abbreviated as SHM). If, in my opinion, the leading position in the creation of new materials is still occupied by physical processes and technologies, then the production of synthetic materials is often more efficient and productive with the help of chemical reactions. And also there was a need to protect materials from corrosion; this, in fact, is the main role of physico-chemical methods in building materials. With the help of physico-chemical methods, physical phenomena that occur during chemical reactions are studied. For example, in the colorimetric method, the color intensity is measured depending on the concentration of the substance; in the conductometric analysis, the change in the electrical conductivity of solutions is measured, etc.
This abstract outlines some types of corrosion processes, as well as ways to combat them, which is the main practical task of physical and chemical methods in building materials.
Physico-chemical methods of analysis and their classification.
Physicochemical methods of analysis (PCMA) are based on the use of the dependence of the physical properties of substances (for example, light absorption, electrical conductivity, etc.) on their chemical composition. Sometimes in the literature physical methods of analysis are separated from FCMA, thereby emphasizing that FCMA uses a chemical reaction, while physical methods do not. Physical methods analysis and physical chemical analysis, mainly in Western literature, are called instrumental, since they usually require the use of instruments, measuring instruments. Instrumental methods of analysis generally have their own theory, different from the theory of methods of chemical (classical) analysis (titrimetry and gravimetry). The basis of this theory is the interaction of matter with the flow of energy.
When using PCMA to obtain information about the chemical composition of a substance, the sample under study is exposed to some type of energy. Depending on the type of energy in a substance, a change occurs in the energy state of its constituent particles (molecules, ions, atoms), which is expressed in a change in one or another property (for example, color, magnetic properties, etc.). By registering a change in this property as an analytical signal, information is obtained about the qualitative and quantitative composition of the object under study or about its structure.
According to the type of disturbance energy and the measured property (analytical signal), FCMA can be classified as follows (Table 2.1.1).
In addition to those listed in the table, there are many other private FHMAs that do not fall under this classification.
Greatest practical use have optical, chromatographic and potentiometric methods of analysis.
Table 2.1.1.
Type of disturbance energy
Property being measured
Method name
Method group name
Electron flow (electrochemical reactions in solutions and on electrodes)
Voltage, potential
Potentiometry
Electrochemical
Electrode polarization current
Voltamperometry, polarography
Current strength
Amperometry
Resistance, conductivity
Conductometry
Impedance (AC resistance, capacitance)
Oscillometry, high-frequency conductometry
Amount of electricity
Coulometry
Electric product weight chemical reaction
Electrogravimetry
The dielectric constant
Dielcometry
Electromagnetic radiation
Wavelength and intensity of the spectral line in the infrared, visible and ultraviolet parts of the spectrum =10-3...10-8 m
Optical methods (IR spectroscopy, atomic emission analysis, atomic absorption analysis, photometry, luminescence analysis, turbidimetry, nephelometry)
Spectral
The same, in the X-ray region of the spectrum =10-8...10-11 m
X-ray photoelectron, Auger spectroscopy
Relaxation times and chemical shift
Nuclear magnetic resonance (NMR) and electron paramagnetic resonance (EPR) spectroscopy
Temperature
Thermal analysis
Thermal
Thermogravimetry
Quantity of heat
Calorimetry
Enthalpy
Thermometric analysis (enthalpimetry)
Mechanical properties
Dilatometry
Energy of chemical and physical (van der Waals forces) interactions
Electrical conductivity Thermal conductivity Ionization current
Gas, liquid, sediment, ion exchange, gel permeation chromatography
Chromatographic
Compared to classical chemical methods, FCMAs are characterized by a lower detection limit, time and labor intensity. FCMAs make it possible to carry out analysis at a distance, automate the analysis process and perform it without destroying the sample (non-destructive analysis).
According to the methods of determination, direct and indirect FCMA are distinguished. In direct methods, the amount of a substance is found by directly converting the measured analytical signal into the amount of a substance (mass, concentration) using the coupling equation. IN indirect methods the analytical signal is used to determine the end of a chemical reaction (as a kind of indicator), and the amount of the analyte that has entered into the reaction is found using the law of equivalents, i.e. according to an equation not directly related to the name of the method.
Based on the method of quantitative determination, a distinction is made between non-reference and reference instrumental methods of analysis.
Without reference methods, they are based on strict laws, the formulaic expression of which makes it possible to recalculate the intensity of the measured analytical signal directly in the amount of the substance being determined using only tabular values. Such a pattern can be, for example, Faraday’s law, which makes it possible to calculate the amount of the analyte in a solution during coulometric titration based on the current and time of electrolysis. There are very few non-standard methods, since each analytical determination is a system of complex processes in which it is impossible to theoretically take into account the influence of each of the numerous operating factors on the result of the analysis. In this regard, certain techniques are used in analyzes that allow these influences to be experimentally taken into account. The most common technique is the use of standards, i.e. samples of substances or materials with precisely known content of the element being determined (or several elements). When carrying out the analysis, the analyte of the test sample and the standard is measured, the data obtained are compared, and the content of this element in the analyzed sample is calculated from the known content of the element in the standard. Standards can be produced industrially(standard samples, normal steels) or prepared in the laboratory immediately before analysis (comparison samples). If chemically pure substances (impurities less than 0.05%) are used as standard samples, then they are called standard substances.
In practice, quantitative determinations using instrumental methods are carried out using one of three ways: calibration function (standard series), standards (comparison) or standard additions.
When working according to the calibration function method, using standard substances or standard samples, a number of samples (or solutions) containing different, but precisely known quantities of the component being determined are obtained. This series is sometimes called the standard series. Then this standard series is analyzed and the sensitivity value K is calculated from the data obtained (in the case of a linear calibration function). After this, the intensity of the analytical signal A in the object under study is measured and the amount (mass, concentration) of the desired component is calculated using the coupling equation /> or found using the calibration graph (see Fig. 2.1.1).
The comparison method (standards) is applicable only for the linear calibration function. The determination of this component is carried out in standard sample(standard substance) and get
Then they are determined in the analyzed object
Dividing the first equation by the second eliminates sensitivity
and calculate the result of the analysis
The method of standard additions is also applicable only to the linear calibration function. In this method, a sample of the test object is first analyzed and // is obtained, then a known amount (mass, volume of solution) of the component being determined is added to the sample and after analysis,
By dividing the first equation by the second, K is eliminated and a formula is obtained for calculating the results of the analysis:
The spectrum of a substance is obtained by influencing it with temperature, electron flow, light flux ( electromagnetic energy) with a certain wavelength (radiation frequency) and other methods. At a certain amount of impact energy, a substance is able to go into an excited state. In this case, processes occur that lead to the appearance of radiation with a certain wavelength in the spectrum (Table 2.2.1).
Emission, absorption, scattering or refraction electromagnetic radiation can be considered as an analytical signal that carries information about the qualitative and quantitative composition of a substance or its structure. The frequency (wavelength) of the radiation is determined by the composition of the substance under study, and the intensity of the radiation is proportional to the number of particles that caused its appearance, i.e. quantity of a substance or component of a mixture.
Each of the analytical methods usually does not use the full spectrum of a substance, covering the wavelength range from X-rays to radio waves, but only a certain part of it. Spectral methods are usually distinguished by the range of spectral wavelengths that are working for this method: ultraviolet (UV), x-ray, infrared (IR), microwave, etc.
Methods that operate in the UV, visible and IR ranges are called optical. They are most used in spectral methods due to the comparative simplicity of the equipment for obtaining and recording the spectrum.
Atomic emission analysis (AEA) is based on the qualitative and quantitative determination of the atomic composition of a substance by obtaining and studying the emission spectra of atoms that make up the substance.
Pi AEA, the analyzed sample of the substance is introduced into the excitation source of the spectral device. In the excitation source, this sample undergoes complex processes consisting of melting, evaporation, dissociation of molecules, ionization of atoms, excitation of atoms and ions.
Excited atoms and ions after a very short time (~10-7-108 s) spontaneously return from an unstable excited state to a normal or intermediate state. This leads to the emission of light with frequency and the appearance of a spectral line.
The general scheme of atomic emission can be represented as follows:
A + E A* A + h
The degree and intensity of these processes depends on the energy of the excitation source (ES).
The most common IWs are: gas flame, arc and spark discharges, inductively coupled plasma (ICP). Their energy characteristic can be considered temperature.
Quantitative AEA is based on the relationship between the concentration of an element and the intensity of its spectral lines, which is determined by the Lomakin formula:
where I is the intensity of the spectral line of the element being determined; c - concentration; a and b are constants.
The values of a and b depend on the properties of the analytical line, IV, and the ratio of the concentrations of elements in the sample, therefore the dependence /> is usually established empirically for each element and each sample. In practice, the method of comparison with a standard is usually used.
For quantitative determinations, the photographic method of recording the spectrum is mainly used. The intensity of the spectral line obtained on a photographic plate is characterized by its blackening:
where S is the degree of blackening of the photographic plate; I0 is the intensity of light passing through the unblackened part of the plate, and I - through the blackened part, i.e. spectral line. The measurement of spectral line blackening is carried out in comparison with the background blackening or in relation to the intensity of the reference line. The resulting blackening difference (S) is directly proportional to the logarithm of the concentration (c):
In the three-standard method, the spectra of three standards with known elemental contents and the spectrum of the analyzed sample are photographed on one photographic plate. The blackening of the selected lines is measured. A calibration graph is constructed from which the content of the elements being studied is determined.
In the case of analyzing objects of the same type, the constant graph method is used, which is built using a large number of standards. Then, under strictly identical conditions, the spectrum of the sample and one of the standards is taken. Using the spectrum of the standard, they check whether the graph has shifted. If there is no shift, then the unknown concentration is found using a constant graph, and if there is, then the magnitude of the shift is taken into account using the spectrum of the standard.
With quantitative AEA, the error in determining the base content is 1-5%, and the impurity content is up to 20%. The visual method of recording the spectrum is faster, but less accurate than the photographic one.
Based on the hardware design, it is possible to distinguish AEAs with visual, photographic and photoelectric registration and measurement of the intensity of spectral lines.
Visual methods (registration with the eye) can only be used to study spectra with wavelengths in the region of 400 - 700 nm. The average spectral sensitivity of the eye is maximum for yellow-green light with a wavelength of 550 nm. Visually, it is possible to establish with sufficient accuracy the equality of the intensities of lines with the nearest wavelengths or determine the brightest line. Visual methods are divided into styloscopic and stylometric.
Styloscopic analysis is based on a visual comparison of the intensities of the spectral lines of the analyzed element (impurity) and nearby spectral lines of the main element of the sample. For example, when analyzing steels, the intensities of the spectral lines of impurities and iron are usually compared. In this case, previously known styloscopic features are used, in which the equality of the intensity of the lines of a certain analytical pair corresponds to a certain concentration of the analyzed element.
Steeloscopes are used for express analysis, which does not require high accuracy. 6-7 elements are determined in 2-3 minutes. The sensitivity of the analysis is 0.01-0.1%. For analysis, both stationary steeloscopes SL-3... SL-12 and portable SLP-1... SLP-4 are used.
Stylometric analysis differs from styloscopic analysis in that the brighter line of the analytical pair is weakened using a special device (photometer) until the intensities of both lines are equal. In addition, stylometers allow the analytical line and the comparison line to be brought closer together in the field of view, which significantly increases the accuracy of measurements. Stylometers ST-1... ST-7 are used for analysis.
The relative error of visual measurements is 1 – 3%. Their disadvantages are the limited visible spectrum, tediousness, and lack of objective documentation of the analysis.
Photographic methods are based on photographic recording of the spectrum using special spectrograph instruments. Workspace spectrographs are limited to a wavelength of 1000 nm, i.e. They can be used in the visible region and UV. The intensity of spectral lines is measured by the degree of blackening of their image on a photographic plate or film.
Basic building materials studied by physical and chemical methods. Construction materials and products used in construction, reconstruction and repair of various buildings and structures, divided into natural and artificial, which in turn are divided into two main categories: the first category includes: brick, concrete, cement, timber etc. They are used in the construction various elements buildings (walls, ceilings, coverings, floors). To the second category - special purposes: waterproofing, thermal insulation, acoustic, etc. The main types of building materials and products are: stone natural building materials from them; binding materials inorganic and organic; forest materials and products made from them; hardware. IN depending on the purpose, conditions of construction and operation of buildings and structures, appropriate building materials are selected, which have certain qualities and protective properties from exposure to them from different external environments. Taking these features into account, any construction the material must have certain construction and technical properties. For example, the material for the external walls of buildings should have the least thermal conductivityIntroduction
Section No. 1. "Building materials and their behavior in fire conditions."
Topic 1. Basic properties of building materials, research methods and assessment of the behavior of building materials in fire conditions.
Topic 2. Stone materials and their behavior in fire conditions.
Topic 3. Metals, their behavior in fire conditions and ways to increase resistance to its effects.
Topic 4. Wood, its fire hazard, fire protection methods and assessment of their effectiveness.
Topic 5. Plastics, their fire hazard, methods of its research and assessment.
Topic 6. Standardization of fireproof use of materials in construction.
Section No. 2. " Building construction, buildings, structures and their behavior in fire conditions.”
Topic 7. Basic information about space-planning and structural solutions of buildings and structures.
Topic 8. Basic information about the fire hazard of buildings and building structures.
Topic 9. Theoretical basis development of methods for calculating the fire resistance of building structures.
Topic 10. Fire resistance of metal structures.
Topic 11. Fire resistance of wooden structures.
Topic 12. Fire resistance of reinforced concrete structures.
Topic 13. Behavior of buildings and structures in fire conditions.
Topic 14. Prospects for improving the approach to determining and regulating fire resistance requirements for building structures.
Introduction
The structure of the discipline, its significance in the process of professional training of institute graduates. Modern directions in design, construction, operation, buildings and structures.
The national economic significance of the activities of fire department employees in monitoring the fire-safe use of building materials and the use of fire-resistant building structures in the design, construction, and reconstruction of buildings and structures.
Section 1. Building materials and their behavior in fire conditions.
Topic 1. Basic properties of building materials, research methods and assessment of the behavior of building materials in fire conditions.
Types, properties, features of production and use of basic building materials and their classification. Factors influencing the behavior of building materials in fire conditions. Classification of the main properties of building materials.
Physical properties and indicators that characterize them: porosity, hygroscopicity, water absorption, water, gas and vapor permeability of building materials.
Basic forms of communication between moisture and material.
Thermophysical properties and indicators characterizing them.
The main negative processes that determine the behavior of inorganic building materials in fire conditions. Methods for experimental assessment of changes in the mechanical characteristics of building materials in relation to fire conditions.
Processes occurring in organic materials under fire conditions. Fire-technical characteristics of building materials, methods of their research and evaluation.
Practical lesson 1. Determination of the basic properties of some building materials and prediction of the behavior of these materials in fire conditions.
Based on the analysis of the optical spectra of atoms and molecules, spectral optical methods for determining the chemical composition of substances have been created. These methods are divided into two: studying the emission spectra of the substances under study (emission spectral analysis); study of their absorption spectra (absorption spectral analysis, or photometry).
When determining the chemical composition of a substance by emission spectral analysis, the spectrum emitted by atoms and molecules in an excited state is analyzed. Atoms and molecules become excited under the influence of high temperatures achieved in a burner flame, in an electric arc or in a spark gap. The radiation obtained in this way is decomposed into a spectrum by a diffraction grating or prism of a spectral device and recorded by a photoelectric device.
There are three types of emission spectra: line, stripe and continuous. Line spectra are emitted by excited atoms and ions. Banded spectra occur when light is emitted by hot pairs of molecules. Continuous spectra are emitted by hot liquids and solids.
Qualitative and quantitative analysis of the composition of the material under study is carried out using characteristic lines in the emission spectra. To decipher spectra, tables of spectral lines and atlases with the most characteristic lines of elements of the Mendeleev periodic system are used. If it is necessary to establish only the presence of certain impurities, then the spectrum of the substance under study is compared with the spectrum of a reference substance that does not contain impurities. The absolute sensitivity of spectral methods is 10 -6 10 -8 g.
An example of the application of emission spectral analysis is the qualitative and quantitative analysis of reinforcing steel: determination of impurities of silicon, carbon, manganese and chromium in the sample. The intensities of the spectral lines in the sample under study are compared with the spectral lines of iron, the intensities of which are taken as the standard.
Optical spectral methods for studying substances also include the so-called flame spectroscopy, which is based on measuring the radiation of a solution introduced into the flame. This method is used to determine, as a rule, the content of alkali and alkaline earth metals in building materials. The essence of the method is that a solution of the test substance is sprayed into the flame zone of a gas burner, where it turns into a gaseous state. Atoms in this state absorb light from a standard source, giving line or striped absorption spectra, or they themselves emit radiation, which is detected by photoelectronic measuring equipment.
The method of molecular absorption spectroscopy allows you to obtain information about the relative position of atoms and molecules, intramolecular distances, bond angles, electron density distribution, etc. In this method, when visible, ultraviolet (UV) or infrared (IR) radiation passes through a condensed substance, partial or complete absorption of radiation energy of certain wavelengths (frequencies). The main task of optical absorption spectroscopy is to study the dependence of the intensity of light absorption by a substance on the wavelength or vibration frequency. The resulting absorption spectrum is an individual characteristic of the substance and, on its basis, qualitative analyzes of solutions or, for example, building and colored glasses are carried out.